Definition of the Isoelectric Point (pI)
The isoelectric point (pI) is scientifically defined as the pH at which a molecule—most commonly a protein, peptide, or amino acid—has no net electrical charge. At this point, the number of positively charged groups (protonated amines, for example) exactly equals the number of negatively charged groups (such as deprotonated carboxyls), resulting in a zwitterionic state where the molecule is neutral in net charge but still possesses internal charges.
This state of electrical neutrality is not just theoretical—it has profound implications for how molecules behave in electric fields, in solution, and during interaction with other charged surfaces or molecules. In electrophoretic systems, for example, a protein at its isoelectric point will cease to migrate under an electric field because it lacks net charge to be influenced by the field. In solution, the electrostatic repulsion between molecules is minimized at the pI, often leading to aggregation or precipitation due to insufficient repulsive forces.
The concept of pI is particularly nuanced for complex biomolecules. For simple amino acids with only two ionizable groups (an amino and a carboxyl group), the pI can be calculated by averaging the pKa values of those groups. However, for larger molecules such as peptides or proteins—often containing multiple ionizable side chains—the calculation becomes significantly more complex and must account for the individual pKa values of all ionizable residues, their local environment, and their interactions.
A protein's isoelectric point is not fixed universally—it can be subtly altered by its conformation, post-translational modifications, or local environment. This variability makes pI a powerful diagnostic marker in proteomics and a critical design parameter in drug development and formulation science.
Relationship Between Isoelectric Point and Molecular Charge
The net charge of a protein or peptide is a direct function of the pH of its surrounding environment. Below its pI, the molecule tends to carry a net positive charge due to protonation of ionizable groups. Above the pI, it carries a net negative charge due to deprotonation. This property plays a pivotal role in electrophoresis, ion-exchange chromatography, and precipitation techniques, where charge-based separations are crucial.
Importance of pI in Protein and Peptide Characterization
The pI is a critical determinant in:
- Protein solubility: Proteins are least soluble at their isoelectric point due to minimal electrostatic repulsion.
- Crystallization conditions: Optimal crystallization often occurs near the pI.
- Purification strategies: pI values guide the selection of chromatographic media and buffer systems.
- Formulation development: Ensures stability and avoids aggregation in therapeutic proteins.
Theoretical Foundation
Amphoteric Electrolytes
Proteins and peptides are amphoteric molecules, meaning they contain both acidic and basic groups. These groups—such as terminal amino and carboxyl groups and ionizable side chains—can accept or donate protons depending on pH, creating a spectrum of charge states.
Henderson-Hasselbalch Equation
The Henderson-Hasselbalch equation is foundational for calculating the pI of molecules with simple structures:
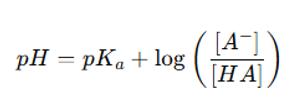
In polyprotic systems such as amino acids with multiple ionizable groups, the isoelectric point is generally estimated by averaging the pKa values that flank the zwitterionic form:
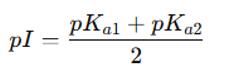
For proteins, which contain multiple ionizable residues, computational approaches are employed to calculate a weighted average of all relevant pKa values.
Influence of Amino Acid Side-Chain pKa on Protein pI
The overall pI of a protein is highly dependent on the composition and distribution of ionizable side chains, including:
- Acidic residues (Asp, Glu): lower the pI
- Basic residues (Lys, Arg, His): raise the pI
- Tyrosine, Cysteine: contribute to finer pI modulation
Post-translational modifications (PTMs) like phosphorylation or glycosylation can shift pI significantly, a feature exploited in proteomics and structural biology.
Methods of Isoelectric Point Determination
Accurate determination of the isoelectric point (pI) is essential for characterizing the charge profile of biomolecules, particularly proteins and peptides. Since the pI directly influences molecular interactions, solubility, and stability, it is a critical quality attribute in both research and industrial settings. Over the years, a variety of analytical techniques have been developed to measure the pI with increasing resolution, throughput, and automation. Each method offers unique advantages, and the choice depends on the specific application, sample complexity, and analytical goals.
Isoelectric Focusing (IEF)
IEF is one of the most classical and widely used techniques for pI determination. It operates on the principle that a charged protein will migrate in an electric field through a medium with a stable pH gradient until it reaches the pH corresponding to its isoelectric point—where its net charge is zero—and then stops migrating.
Principle: Proteins are separated according to their pI within a polyacrylamide gel or immobilized pH gradient strip containing ampholytes. Upon application of an electric field, proteins migrate to and focus at their pI.
Operational Steps:
1. Prepare a gel strip or gel bed containing a preformed or generated pH gradient.
2. Load protein samples into the medium.
3. Apply an electric field to initiate migration.
4. Allow proteins to focus and separate based on pI.
5. Visualize the protein bands using staining techniques (e.g., Coomassie, silver stain).
Advantages:
- High resolution for complex protein mixtures.
- Can separate proteins differing by as little as 0.01 pH units.
- Suitable for identifying charge variants in biopharmaceuticals.
Limitations:
- Labor-intensive and time-consuming.
- Limited quantification accuracy.
- Gels can be fragile and prone to variability.
Capillary Isoelectric Focusing (cIEF)
Capillary isoelectric focusing (cIEF) is a modern, miniaturized form of IEF performed within a capillary, offering enhanced sensitivity, reproducibility, and automation. It uses UV or fluorescence detection and is commonly used in regulated environments for biopharmaceutical product characterization.
Principle: Proteins are mixed with carrier ampholytes and injected into a narrow capillary. An electric field is applied, and molecules migrate to their pI. Once focused, the pH gradient is fixed by chemical mobilization, and the proteins are detected at the capillary outlet.
Advantages:
- Superior resolution compared to traditional gel IEF.
- High sensitivity (nanogram to picogram level).
- Low sample consumption.
- Automation-compatible, making it ideal for high-throughput and QC settings.
Limitations:
- Requires precise pH calibration and optimization.
- Can be sensitive to sample impurities or ampholyte composition.
Imaging Capillary IEF (icIEF)
Imaging capillary isoelectric focusing (icIEF) represents an evolution of cIEF by integrating imaging-based detection systems that allow real-time monitoring of the focusing process within the capillary.
Key Features:
- Simultaneous multi-point UV detection across the capillary.
- Improved accuracy for quantifying charge variants.
- Real-time data acquisition and pI profile visualization.
Applications:
- Characterization of monoclonal antibodies and biosimilars.
- Charge variant profiling under stress or degradation conditions.
- Lot-to-lot consistency monitoring in biologics manufacturing.
Advantages over cIEF and traditional IEF:
- Eliminates mobilization steps, reducing variability.
- Higher data integrity and reproducibility.
- Widely accepted in regulatory filings.
Liquid Chromatography–Mass Spectrometry (LC-MS/MS)
Although LC-MS/MS does not directly measure the isoelectric point, it plays a vital complementary role by enabling detailed characterization of molecular features that influence pI, such as post-translational modifications (PTMs), sequence variants, and truncations.
Applications in pI Analysis:
- Identification of phosphorylation, deamidation, or glycosylation events that alter net charge.
- Confirmation of structural variants seen in IEF or icIEF.
- pKa prediction through computational modeling using sequence and structure data.
Advantages:
- Unparalleled specificity and structural insight.
- Can resolve molecular heterogeneity undetectable by IEF alone.
- Enables bottom-up and top-down proteomic analysis.
Limitations:
- Indirect estimation of pI.
- Requires significant technical expertise and instrumentation.
Applications of Isoelectric Point in Protein Research
Protein Purification and Separation
Ion-Exchange Chromatography (IEX)
Ion-exchange chromatography is one of the most commonly used techniques for protein purification. The pI of a protein dictates its net charge at any given pH, and this principle is used to modulate its binding to cation or anion exchange resins. For instance:
- If the buffer pH is below the pI, the protein carries a net positive charge and binds to cation exchange resins.
- If the buffer pH is above the pI, the protein carries a net negative charge and binds to anion exchange resins.
Knowing the pI allows precise pH adjustment for selective binding or elution, enhancing purification yield and specificity.
Precipitation-Based Separation
Proteins are least soluble at their isoelectric point, as electrostatic repulsion is minimized. This property is leveraged in salting-out and isoelectric precipitation techniques, which help concentrate or fractionate protein mixtures by adjusting pH to near the target protein's pI.
Protein Stability and Solubility
pH-Dependent Solubility
Protein solubility typically exhibits a U-shaped profile as a function of pH, with the lowest solubility at the pI. Operating at pH values away from the pI helps maintain proteins in solution by maximizing electrostatic repulsion, which is critical for preventing aggregation.
Protein Aggregation Behavior
At the isoelectric point, protein molecules lack repulsive electrostatic forces and tend to self-associate, increasing the risk of aggregation. This is especially relevant in formulation studies and structural stability assessments. Monitoring and modulating the pI can help prevent aggregation during storage, transport, and processing.
Protein Crystallization
Protein crystallization is highly sensitive to charge distribution. Near the isoelectric point, reduced electrostatic repulsion can facilitate the ordered packing of protein molecules, a prerequisite for crystal lattice formation. Thus, many crystallization protocols deliberately target pH conditions close to the pI to enhance success rates in X-ray crystallography.
Service we provide:
- Protein Post-translational Modification Analysis
- Protein-Protein Interaction Analysis Service
- Protein Gel and Imaging
- Protein Identification Services
- Protein Quantification
Role of Isoelectric Point in Drug Development
Protein Drug Formulation Optimization
Therapeutic proteins (e.g., monoclonal antibodies, enzymes, cytokines) must maintain stability and bioactivity over extended storage durations. The pI informs buffer selection and excipient compatibility, ensuring that the formulation pH remains sufficiently distant from the pI to avoid:
- Aggregation
- Precipitation
- Denaturation
By designing formulations with a pH at least one unit above or below the protein's pI, developers can extend product stability and reduce immunogenicity risks.
Drug–Target Electrostatic Compatibility
Electrostatic interactions are a primary force driving drug–target recognition, especially for protein–protein and protein–ligand binding. The pI provides valuable input for molecular modeling and docking studies, enabling:
- Prediction of interaction hotspots
- Optimization of binding affinity through charge engineering
- Avoidance of off-target effects driven by charge-based nonspecific interactions
This makes pI profiling an important element in rational drug design pipelines.
Biologics Development and Characterization
In therapeutic monoclonal antibody development, pI serves as a critical quality attribute (CQA). Charge heterogeneity, often revealed through shifts in pI, can result from deamidation, glycation, or other modifications and may affect:
- Efficacy
- Pharmacokinetics
- Immunogenicity
Techniques such as icIEF are routinely used in the QC and regulatory qualification of biologics to detect and quantify charge variants, ensuring batch-to-batch consistency.
Biosimilar Development
For biosimilar candidates to gain approval, they must demonstrate analytical similarity to the originator product. Matching pI profiles through high-resolution methods like cIEF or icIEF is a fundamental part of the comparability exercise, supporting regulatory submissions and market entry.
Technical Advantages of Modern pI Analysis Services
The evolution of isoelectric point (pI) analysis technologies has transformed what was once a labor-intensive, semi-quantitative process into a highly precise, reproducible, and scalable analytical service. Today's advanced pI analysis platforms deliver not only scientific insight but also critical regulatory and commercial value across biopharmaceutical development pipelines.
High Resolution and Accuracy
Modern methods such as imaging capillary isoelectric focusing (icIEF) and high-resolution cIEF offer exceptional resolution, capable of distinguishing protein variants that differ in pI by as little as 0.02 pH units. This level of granularity is crucial for:
- Detecting subtle charge heterogeneities due to post-translational modifications (PTMs) like deamidation, sialylation, or glycation.
- Ensuring lot-to-lot consistency in biologics manufacturing.
- Supporting detailed comparability assessments for biosimilar development.
These techniques also provide highly accurate pI assignments, typically with standard deviations of less than ±0.1 pH units—well within regulatory requirements for analytical precision.
High-Throughput and Automation-Ready
Cutting-edge pI analysis services are optimized for throughput and scalability, enabling the processing of dozens to hundreds of samples per day. This is particularly beneficial during:
- Clone screening in cell line development
- Formulation screening during drug product optimization
- Stability studies under ICH guidelines
Automated systems reduce hands-on time, minimize variability, and ensure data traceability—key advantages in Good Manufacturing Practice (GMP) and regulated environments.
Multi-Platform Integration
Modern service providers often integrate pI analysis with orthogonal techniques, such as:
- LC-MS/MS for structural elucidation
- Ion-exchange chromatography (IEX) for preparative purification and charge variant isolation
- Differential scanning calorimetry (DSC) or dynamic light scattering (DLS) for thermal and aggregation stability studies
This multi-dimensional analytical approach enhances the interpretability and reliability of pI data within broader developability assessments.
References
Verma, Ravi Kumar, Neel Duti Prabh, and Ramasubbu Sankararamakrishnan. "New subfamilies of major intrinsic proteins in fungi suggest novel transport properties in fungal channels: implications for the host-fungal interactions." BMC evolutionary biology 14 (2014): 1-16. https://doi.org/10.1186/s12862-014-0173-4