Glycans, complex carbohydrate structures, are integral to the biological functions of glycoproteins and glycolipids. Their intricate roles in cellular processes and therapeutic applications underscore the importance of understanding their various types and control ranges. This knowledge is particularly crucial for optimizing glycoprotein-based therapeutics, such as monoclonal antibodies, where glycan modifications can significantly impact drug efficacy and safety. By exploring the common types of glycans and their control ranges, as well as the factors influencing their heterogeneity, we gain insights into how these modifications can be managed to enhance the quality and effectiveness of glycoprotein therapeutics.
Common Types of Glycans and Their Control Ranges
Glycans are structurally complex carbohydrate molecules that play critical roles in the biological functions of glycoproteins and glycolipids. Understanding the common types of glycans and their control ranges is essential for optimizing and ensuring the quality of glycoprotein-based therapeutics, including monoclonal antibodies.
Fucose
Fucose is a common glycan modification in glycoproteins, typically controlled within a range of 2% to 13%. This range reflects the variability in fucosylation levels that can occur during glycoprotein synthesis, influenced by the expression system and other factors.
Galactose
Galactose modification is another prevalent glycan structure in glycoproteins, with a typical control range for galactosylation between 10% and 40%. This variability indicates the degree of galactosylation, which can affect the stability, efficacy, and immunogenicity of the glycoprotein.
Sialic acid, which plays a role in extending glycan chains and influencing glycoprotein charge, is usually controlled within a range of 0% to 2%. The extent of sialylation can impact the glycoprotein's biological activity and its interaction with cellular receptors.
Mannose
High-mannose structures are characterized by an increased mannose content compared to typical glycan profiles, with control ranges generally maintained between 0% and 10%. Variations in mannose content can affect the glycoprotein's folding and function.
The Process of N-Glycan Formation in CHO Cell-Expressed Proteins
N-glycan formation in proteins expressed in Chinese hamster ovary (CHO) cells involves a series of intricate biochemical processes primarily occurring in the endoplasmic reticulum (ER) and Golgi apparatus. This article delineates the key steps involved in the synthesis of N-glycans, emphasizing the roles of various precursor molecules and enzymes.
1. Synthesis in the Endoplasmic Reticulum
The initial stages of N-glycan synthesis occur in the ER, where specific glycosylation processes take place.
Precursor Formation: On the surface of the ER, GDP-mannose (GDP-Man) and UDP-N-acetylglucosamine (UDP-GlcNAc) are utilized to synthesize a lipid-linked oligosaccharide precursor. This precursor, known as GlcNAc₂Man₅, consists of two N-acetylglucosamine (GlcNAc) residues and five mannose (Man) residues.
Oligosaccharide Elongation: Within the ER, the GlcNAc₂Man₅ precursor is further elongated by the addition of three glucose (Glc) residues and four mannose residues, resulting in the formation of Glc₃-GlcNAc₂Man₉.
2. Transfer and Processing in the Golgi Apparatus
Following initial synthesis in the ER, the glycosylation process continues in the Golgi apparatus, where additional modifications occur.
Attachment to Antibody: The oligosaccharide Glc₃-GlcNAc₂Man₉ is transferred to the asparagine residue at position Asn297 of the antibody. This step is facilitated by various glycosyltransferases and involves the removal of three glucose residues and one mannose residue.
Final Modifications: The glycosylated antibody, now carrying GlcNAc₂Man₈, enters the Golgi apparatus. Within the Golgi, high-mannose residues are trimmed, and additional oligosaccharides, including fucose, sialic acid, galactose, and N-acetylglucosamine, are added. This process culminates in the formation of the mature N-glycan structure.
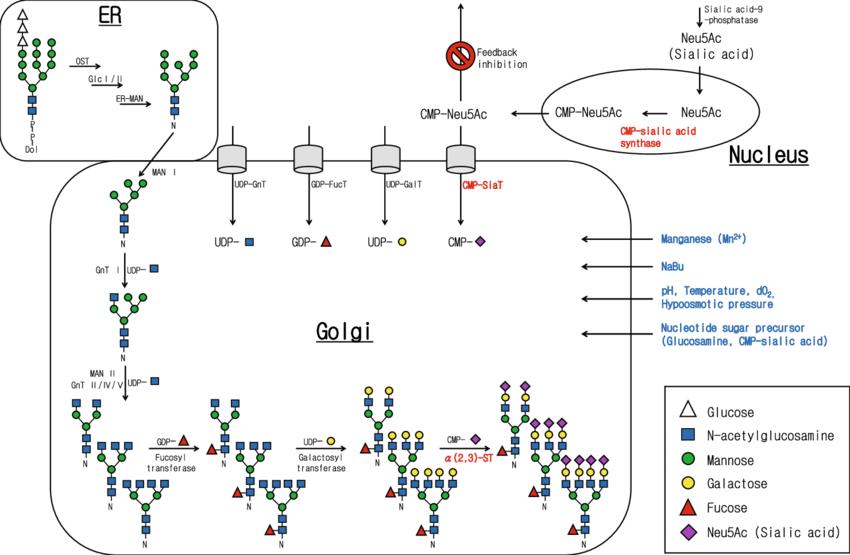
Causes of Glycan Heterogeneity
Glycan heterogeneity in glycoproteins, including monoclonal antibodies, arises from several factors influencing the structure and composition of N-glycans. The primary causes of glycan heterogeneity are outlined below:
1. Core Glycan Composition
The core of N-glycans is composed of GlcNAc and mannose. Additional glycan modifications, such as sialic acid, fucose, and galactose, are typically added to this core structure, forming complex bi-antennary structures. Variations in the addition of these modifications contribute to glycan heterogeneity.
2. Glycan Modifications and Antibody Production
During the synthesis of monoclonal antibodies, variations in glycan modifications are common. N-glycans represent approximately 1% to 3% of the total mass of an antibody. Common issues include the loss of sialic acid, fucose, and galactose, as well as incomplete mannose removal leading to high-mannose variants. These variations are significant contributors to the formation of glycan heterogeneity.
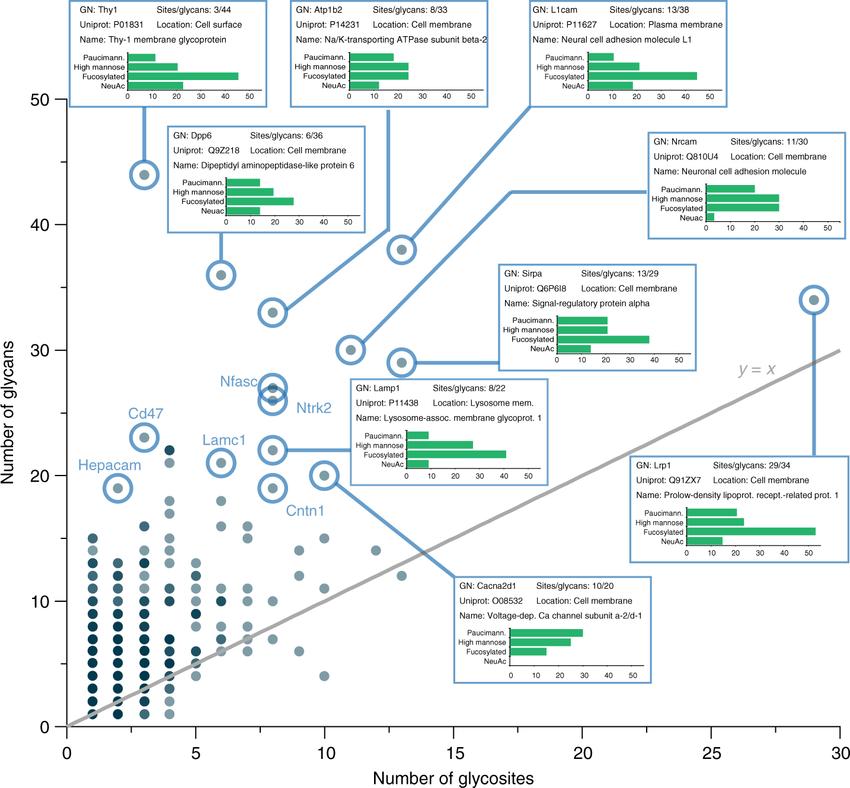
Effects of Glycan Heterogeneity on Drug Efficacy
Glycosylation modifications are critical quality attributes (CQA) in the production of antibody drugs and have substantial effects on drug efficacy and pharmacokinetics. The impacts of glycan heterogeneity are summarized below:
1. Antibody-Dependent Cellular Cytotoxicity (ADCC)
Antibodies lacking fucose modifications exhibit enhanced ADCC activity. This increased activity is due to the improved binding affinity of fucosylated antibodies to Fcγ receptors on immune cells.
2. Complement-Dependent Cytotoxicity (CDC)
The presence of galactose modifications can enhance CDC activity. This effect is attributed to the improved complement activation and subsequent cytotoxic response.
3. Sialic Acid Modifications
Sialic acid modifications generally reduce ADCC activity but extend the in vivo half-life of the antibody. This extended half-life results from reduced recognition and clearance by the immune system.
4. High-Mannose Modifications
Antibodies with high-mannose modifications often have a reduced half-life. High-mannose glycans can lead to accelerated clearance from the bloodstream due to altered interactions with cellular receptors.
5. N-Acetylglucosamine Modifications
Glycans with N-acetylglucosamine modifications can enhance ADCC activity. However, this modification may also reduce the drug's overall half-life due to increased uptake and metabolism by cells.
Methods for Controlling Glycan Heterogeneity
Managing glycan heterogeneity in glycoproteins is paramount for their consistent production and therapeutic efficacy. The following methodologies are employed to control glycan heterogeneity:
Selection of Host Cells The choice of host cells is pivotal as different cell lines exhibit varied capacities for post-translational modifications. Selecting an appropriate host cell that produces the desired glycosylation profile can significantly enhance the consistency and quality of glycan structures on proteins.
pH Regulation Extracellular pH influences glycan modifications by altering the pH within the Golgi apparatus, thereby affecting the activity of glycosylation-related enzymes. The impact of pH on glycan profiles, such as galactosylation, varies depending on the cell line and culture conditions. Precise pH control is essential for maintaining consistent glycosylation.
Dissolved Oxygen Levels Dissolved oxygen levels primarily affect the abundance of galactose residues through two mechanisms:
Precursor Availability: Low dissolved oxygen conditions reduce the precursor molecule, UDP-galactose (UDP-Gal).
Redox Potential: Changes in dissolved oxygen levels can alter the redox potential, affecting disulfide bond formation in antibodies. This alteration creates spatial hindrance for the addition of galactose residues, thus impacting galactosylation levels.
Culture Media and Additives The composition of the culture medium and the inclusion of specific supplements can influence glycan structures:
Precursor Supply: The inclusion of glycan precursors such as glucose, galactose, mannose, amino sugars, and N-acetylglucosamine is essential for glycan synthesis.
Supplementation: Elements like calcium, magnesium, manganese, and copper can affect the availability and utilization of glycan precursors, thereby influencing glycan heterogeneity.
Osmotic Pressure and Cultivation Temperature Regulating the osmotic pressure of the culture medium and the temperature during cultivation are crucial factors. These parameters can affect the overall glycosylation process, impacting the stability and composition of glycan structures.
You may interested in
Effects of Common Additives on Glycan Structures
Additives employed during cell culture and protein production can exert substantial effects on glycan structures. The following points elucidate how specific additives impact glycan biosynthesis:
Glucose Concentration
The concentration of glucose significantly influences glycan synthesis. At low glucose levels (0-25 mM), glucose is predominantly utilized by cells as a nutrient for survival rather than as a precursor for glycosylation. This utilization results in decreased overall glycosylation levels and an increased incidence of abnormal glycosylation structures.
D-Galactose
The introduction of D-galactose elevates the intracellular concentration of UDP-galactose (UDP-Gal) while reducing UDP-glucose (UDP-Glc). This alteration enhances the galactosylation of glycoproteins.
Amino Sugars
The incorporation of amino sugars, such as glucosamine (GlcN) or N-acetylglucosamine (GlcNAc), increases intracellular levels of UDP-N-acetylglucosamine (UDP-GlcNAc). This augmentation enhances mannosylation and reduces the structural complexity of glycans.
N-Acetylmannosamine
The addition of N-acetylmannosamine has been demonstrated to facilitate an increase in high mannose structures within glycoproteins.
N-Acetylglucosamine and Other Additives
The inclusion of N-acetylglucosamine, along with other additives such as galactose, uridine, manganese ions (Mn²⁺), and dexamethasone, can enhance the sialylation of protein products at their termini.
Manganese Ions
Manganese ions (Mn²⁺) contribute to an increase in non-fucosylated glycan structures.
Ammonia
Ammonia plays a critical role in glycosylation regulation. Elevated ammonia levels can lead to an increase in the pH of the Golgi apparatus, thereby influencing the transport and utilization of UDP-GlcNAc and sialic acid.
Impact of Temperature on Glycan Regulation
Temperature exerts a significant influence on the regulation of glycan structures in glycoproteins. The following points delineate how temperature affects glycan biosynthesis and regulation:
Enzyme Activity
Temperature impacts glycan structures primarily by affecting the activity of key glycosylation enzymes. The enzymatic activity necessary for glycan formation is temperature-dependent, and deviations from optimal temperatures can alter enzyme efficiency.
Sialic Acid and Galactose Content
Cultivation at lower temperatures significantly reduces the levels of sialic acid and galactose in glycoproteins. This is likely due to the inhibition of enzymatic processes responsible for the addition of these saccharides at reduced temperatures.
Glycosylation Modifications Under Varying Glutamine Concentrations
Under different initial concentrations of glutamine, a reduction in temperature can lead to decreased galactosylation, while sialylation levels may increase. These alterations in glycosylation patterns are contingent upon the specific conditions of the cultivation environment.
Cellular Metabolic Changes
Cooling can induce changes in the cellular metabolic state, affecting the availability of nucleotide sugar donors within the cell. This reduction in donor availability subsequently impacts the glycosylation levels of the Fc region of antibodies.
Considerations for Process Development
During the development phase, it is essential to carefully consider the multifaceted effects of temperature on protein yield and quality. Temperature influences not only the quantity but also the quality of protein expression, making it crucial to evaluate temperature effects when optimizing cultivation processes.
Impact of Expression Systems on Post-Translational Modifications of Glycoproteins
The PTMs of glycoproteins are significantly influenced by the expression systems used. The following points highlight how different expression systems affect glycoprotein glycosylation and other modifications:
1. Variability in Protein Modification
The extent of protein modification varies among different host cells. Selecting an appropriate host cell and, if necessary, a specific clone is crucial. Commonly used host cells for antibody production include Chinese Hamster Ovary (CHO), Baby Hamster Kidney (BHK), NS0, and SP2/0 cells. Among these, CHO cells are the most widely utilized.
2. Glycosylation in NS0 and SP2/0 Cells
Proteins secreted by NS0 and SP2/0 cells often exhibit higher levels of galactosylation and NGNA. These modifications can lead to increased immunogenicity in vivo.
3. Sialic Acid Structures in CHO and BHK Cells
CHO and BHK cells lack 2,6-sialyltransferase, resulting in the presence of only 2,3-sialic acid structures on proteins.
4. Enzyme Deficiencies in CHO Cells
CHO cells are deficient in β-1,4-N-acetylglucosaminyltransferase or related enzymes. This deficiency impacts the glycosylation patterns of proteins expressed in these cells.
5. Considerations for Biosimilars and Novel Drugs
Different cell lines exhibit variations in sialylation, galactosylation, high-mannose content, and fucosylation levels. For biosimilars, it is essential to select host cells with glycosylation patterns similar to the reference drug. For novel drugs, the impact of the cell line's glycosylation modifications on antibody quality should be the primary consideration.
Role of Dopamine in Glycan Regulation
Dopamine's influence on glycan regulation is an area of ongoing research. The following points summarize current understanding of how dopamine and related factors affect glycosylation processes:
1. Impact of Dissolved Oxygen on Galactose Residues
Dissolved oxygen levels primarily affect the galactose residue levels in glycoproteins. The mechanism involves two main factors:
Precursor Availability: Under low dissolved oxygen conditions, the levels of UDP-Gal, a precursor for galactose, decrease.
Redox Potential: Both high and low dissolved oxygen conditions can alter the redox potential, which in turn affects the formation of disulfide bonds in antibodies. These disulfide bonds create steric hindrance, thereby reducing the modification of galactose residues.
2. Effect of Dissolved Oxygen on Charge Heterogeneity
Research has shown that, within a broad range of dissolved oxygen levels (40% to 100%), the charge heterogeneity of secreted proteins in CHO cells remains relatively consistent. Moreover, the levels of glycosylation variants such as G0 and G1 do not exhibit significant changes. Consequently, when optimizing and developing cell culture processes, a comprehensive consideration of dissolved oxygen levels is advisable.
Influence of Osmotic Pressure on Glycan Heterogeneity Regulation
Osmotic pressure in cell culture media plays a significant role in the regulation of glycan heterogeneity. The following points outline the effects of osmotic pressure on glycosylation patterns:
1. Osmotic Pressure in Cell Culture Media
The osmotic pressure of basal cell culture media typically ranges from 280 to 320 mOsm/kg. However, the addition of supplements increases the osmotic pressure of the culture medium. Osmotic pressure affects glycosylation in several ways:
2. Effect of Sodium Chloride Addition in CHO Cell Cultures
Studies have reported that the addition of sodium chloride (NaCl) in CHO cell cultures leads to the following changes:
High-Mannose Glycosylation: As osmotic pressure increases, the level of high-mannose glycosylation (Man5) increases progressively.
G0F and G1F Glycosylation: The content of G0F decreases with increasing osmotic pressure, whereas the levels of G1F remain relatively unchanged.
3. Mechanism Behind Osmotic Pressure Effects on Man5 Levels
The impact of osmotic pressure on Man5 levels may be attributed to high osmotic pressure conditions that reduce the activity of GnT-I (N-acetylglucosaminyltransferase I) and decrease mRNA translation, leading to altered glycosylation patterns.
Conclusion
In conclusion, the regulation of glycan structures in glycoproteins involves a complex interplay of biological processes, including the control of glycan types and their modification ranges. Understanding these factors—ranging from common glycan structures like fucose, galactose, and sialic acid to the effects of additives, temperature, and expression systems—provides essential insights for optimizing glycoprotein-based drugs. The management of glycan heterogeneity is crucial for ensuring consistent therapeutic quality and efficacy. Advances in process control and an in-depth understanding of glycan biosynthesis will continue to drive improvements in the development and production of high-quality glycoprotein therapeutics, ultimately benefiting patient outcomes and therapeutic efficacy.