Introduction to ADC Therapies
Since the inception of cytotoxic agents in cancer therapy, oncologists have relentlessly pursued methods to enhance therapeutic efficacy while minimizing overall patient toxicity. In pursuit of greater tumor cell killing potential, researchers have explored the use of cytotoxic agents with potency at picomolar or lower levels, such as microtubule inhibitors and DNA alkylating agents. However, due to the narrow therapeutic windows of these compounds, they have not been successfully employed in cancer treatment.
With the advent of monoclonal antibodies, leveraging their strong specificity for targeted binding, the creation of antibody-drug conjugates (ADCs) through chemical conjugation of cytotoxic effectors has provided the possibility of selectively delivering cytotoxic drugs to cancer cells. Though translating this "simple concept" into clinical practice has encountered numerous challenges, since the approval of brentuximab vedotin (BV) in 2011 and ado-trastuzumab emtansine (T-DM1) in 2013 by the United States Food and Drug Administration (FDA), the field has witnessed significant progress. Presently, there are at least dozens of ADC candidate drugs undergoing clinical evaluation worldwide.
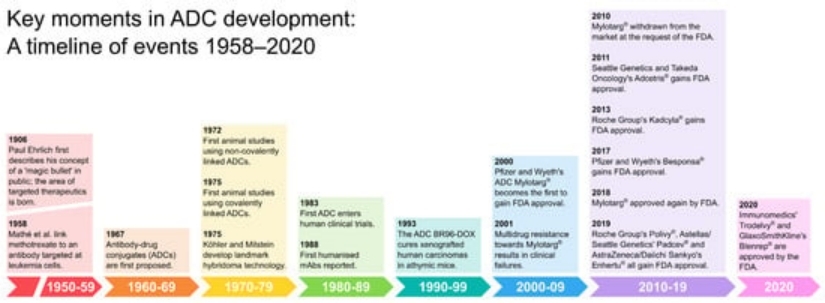
ADC Therapies Background Overview
Molecular Structure and Mechanism of Action of ADCs
ADC molecules consist of monoclonal antibodies, linkers, and payloads (Figure 2).
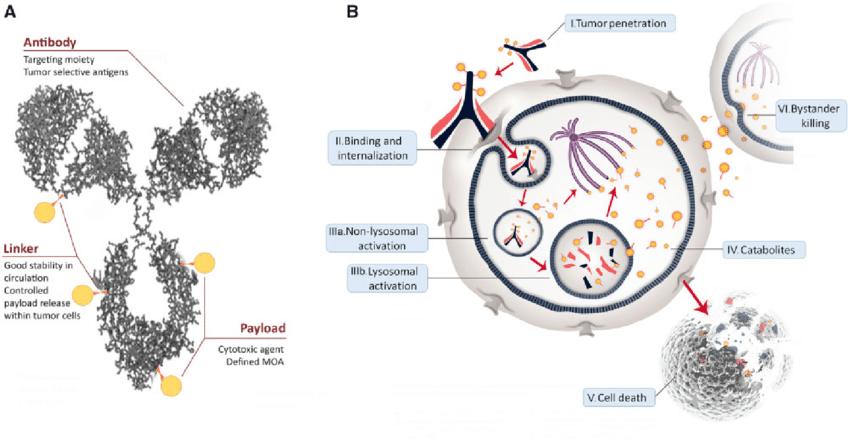
Figure 3 provides a detailed depiction of the core mechanism of action of ADC drugs. ADC molecules enter tumor tissues via the vascular system and tightly bind to specific targets on the surface of tumor cells. Subsequently, internalization is achieved through the endosomal-lysosomal pathway, during which the linker is cleaved or the antibody is degraded, thereby releasing the payload. These payloads eventually diffuse into the cytoplasm and localize to their targets (microtubules), disrupting microtubule dynamics. It is noteworthy that other commonly used payloads, such as calicheamicin, target DNA and require further diffusion into the nucleus from the cytoplasm, a process not depicted in Figure 3.
If cytotoxic metabolites can freely traverse the cell membrane and diffuse, they can enter neighboring cells and exert cytotoxic effects through bystander effects.
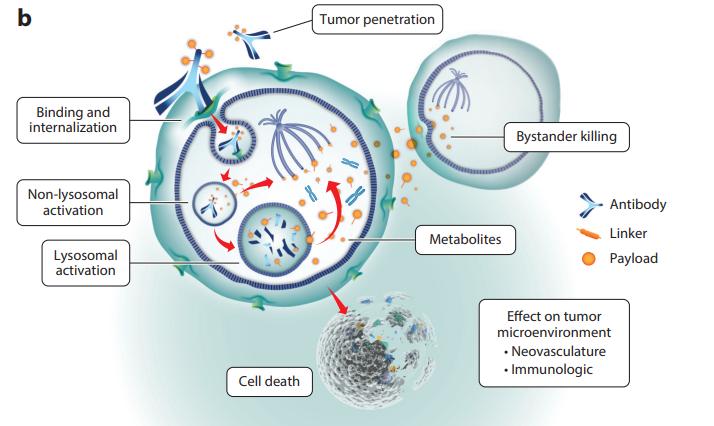
Market of ADC Therapies
The first ADC Therapies worldwide, Mylotarg®, received FDA marketing approval in 2000. Since 2019, the number of ADCs approved for marketing has more than doubled, with a total of 5 ADCs receiving marketing approval between 2019 and 2020.
Table 1 outlines the ADC drugs approved for marketing globally as of 2020 [4].
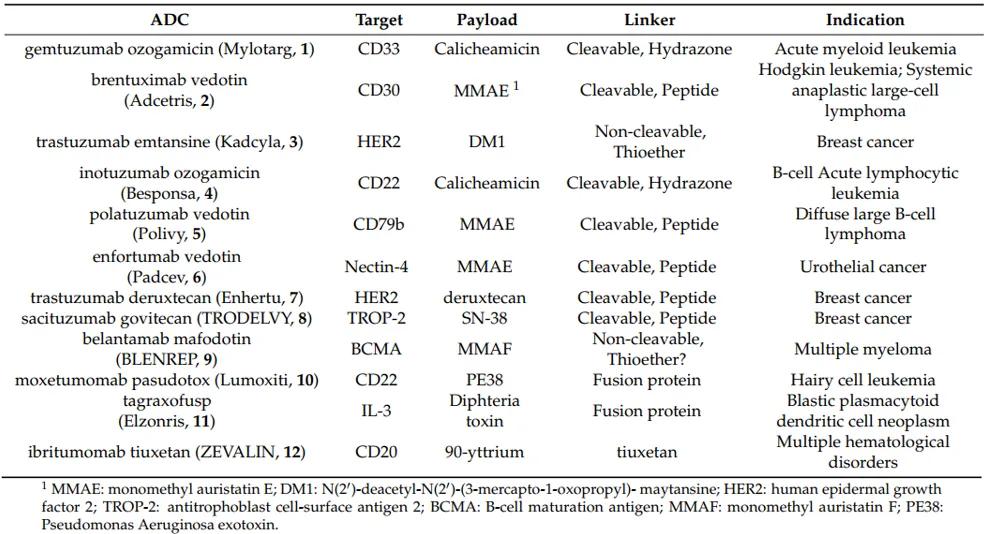
Throughout the global health-care industry, the sale of Antibody-Drug Conjugates (ADCs) currently available in the market has demonstrated an encouraging trajectory of growth across various regions. In an assessment of the market from 2016 to 2020, the sales of ADC pharmaceuticals in the United States surged from $329 million to $1.47 billion, signifying an impressive compound annual growth rate (CAGR) of 45%. Concurrently, the European and Japanese markets for ADC medications also exhibited positive CAGRs of 13% and 21%, respectively.
Mylotarg® (gemtuzumab ozogamicin) stands as the pioneering globally marketed ADC drug, co-developed by Pfizer and Wyeth. It obtained accelerated FDA approval as early as 2000 for the treatment of acute myeloid leukemia in patients experiencing a first relapse, aged 60 years and older, with CD33 expression, and who are not suitable for cytotoxic chemotherapy. Shortly after its market debut, it was voluntarily withdrawn from the market in June 2010 due to safety concerns. Subsequent updates to clinical data and adjustments to specifications culminated in Mylotarg®'s re-approval for marketing by the FDA in 2017. After 17 years of refinement, with the dosage regimen adjusted from the original 9 mg/m2 to 3 mg/m2, this ADC earned its place back on the market [4,5].
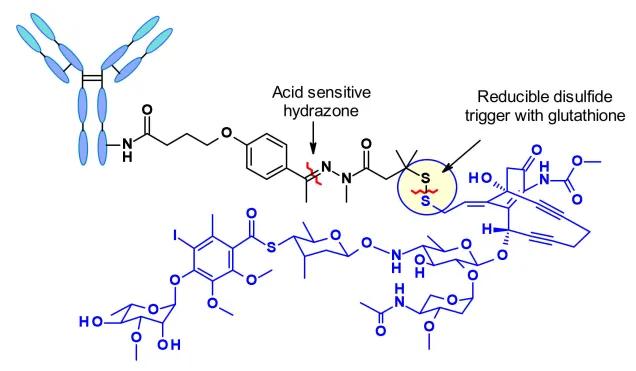
Kadcyla® (ado-trastuzumab emtansine), a remarkable product of a collaborative development endeavor by Roche and ImmunoGen, was officially approved by the Food and Drug Administration (FDA) on the 22nd of February, 2013. This approval marked a significant milestone in the field of oncology as it specifically targets the treatment of human epidermal growth factor receptor 2 (HER2)-positive metastatic breast cancer, following initial treatments with Herceptin® and taxane chemotherapy. Notably, Kadcyla® bears the distinction of being the pioneer ADC drug approved globally for the management of solid tumors.
Characterized as a sophisticated, second-generation ADC, Kadcyla® is formulated with humanized monoclonal antibodies and highly potent cytotoxic drugs. This synergistic combination facilitates a decrease in immunogenicity while simultaneously augmenting the function of the cytotoxic agent. Empirical evidence from clinical trials attests to the drug's efficacy, indicating a significant increase in median progression-free survival (PFS) – 9.6 months - and overall survival (OS) – 29.9 months versus 25.9 months – in patients receiving Kadcyla® as compared to those in the control group.
In another trailblazing achievement, Kadcyla® secured approval in China in January 2020. This marked the inaugural approval of an ADC drug within the Chinese territory. Bolstering its commendable reputation, Kadcyla® achieved a record-breaking global sale of $2.18 billion in 2021, positioning itself as the leading ADC drug in the market [4,5]
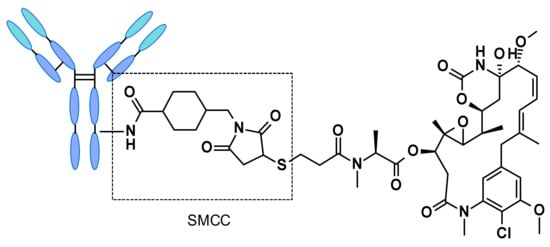
Pharmacological Studies of ADC Therapies
The structure of ADC molecules is intricate and multifaceted. Designing these molecules requires careful consideration of various factors and their interrelationships, including antigen selection, antibody design, payload selection, linker design, and conjugation strategies [7]. Refer to Figure 6 for a detailed overview of specific considerations.
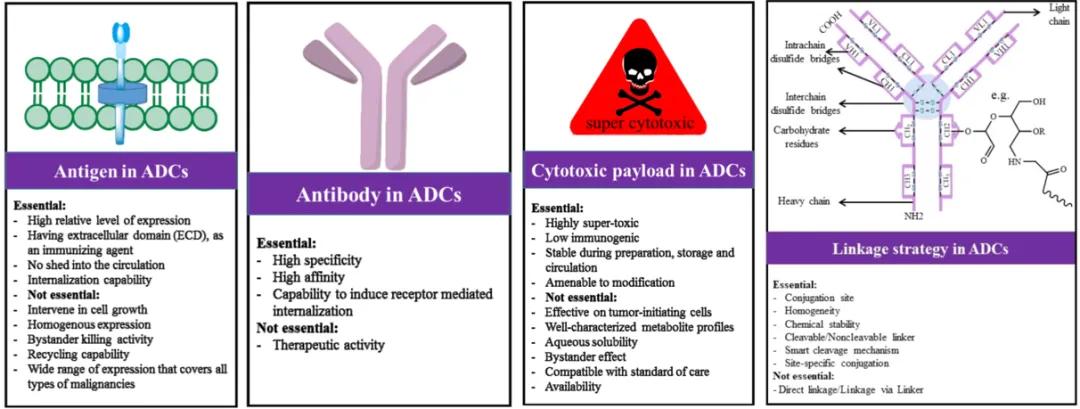
Cytotoxic Payloads
The cytotoxic payload is a critical component of ADCs responsible for exerting cytotoxic effects, necessitating several key requirements: (1) high cytotoxicity, typically with IC50 values at low nanomolar or picomolar levels (Figure 7); (2) well-defined targets and mechanisms of action; (3) (potential) chemical attachment sites.
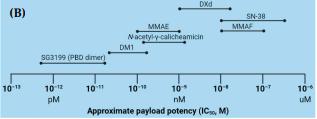
Derivatives of maytansine (DM1/DM4) or auristatins (MMAE/MMAF) are commonly used microtubule inhibitors. Other classes of cytotoxic agents include enediynes (calicheamicin), anthracycline derivatives, pyrrolobenzodiazepines (PBDs), and indolocarbazoles, all targeting DNA minor grooves. Additionally, camptothecin alkaloids (SN-38) inhibit DNA topoisomerase I.
Among the 114 completed or ongoing clinical trials, there is a lack of diversity in the cytotoxic drug payloads utilized, with only 7 reported payload formulations (4 trials are ongoing with undisclosed structures). Six out of the seven payload mixtures are derived from natural sources, underscoring the pivotal value of natural products in the repertoire of ADC cytotoxic payloads [7,8].
You may interested in
Linkers
One of the foremost challenges in ADC development lies in the selection of appropriate linkers to conjugate cytotoxic payloads with antibodies. The chemistry of linkers influences various properties of ADCs, including toxicity, specificity, stability, and potency. Common linker technologies and release mechanisms employed in ADCs are detailed in Table 2.
Table 2. ADC linker technologies and release mechanisms
Linker | Release Mechanism |
Disulfide | Designed to be cleaved via thiol exchange with intracellular thiols such as glutathione |
Hydrazone | Designed for serum stability and degradation within acidic intracellular compartments |
Peptide | Designed to be hydrolyzed by lysosomal proteases such as cathepsin B |
Thioether | Irreducible, specifically designed for intracellular proteolytic degradation |
The linkers can be broadly categorized into cleavable type, where the payload can detach from the monoclonal antibody at the tumor site, or non-cleavable type, where the payload remains bound to the monoclonal antibody, which undergoes degradation after internalization. Please refer to Figure 8 for illustration.
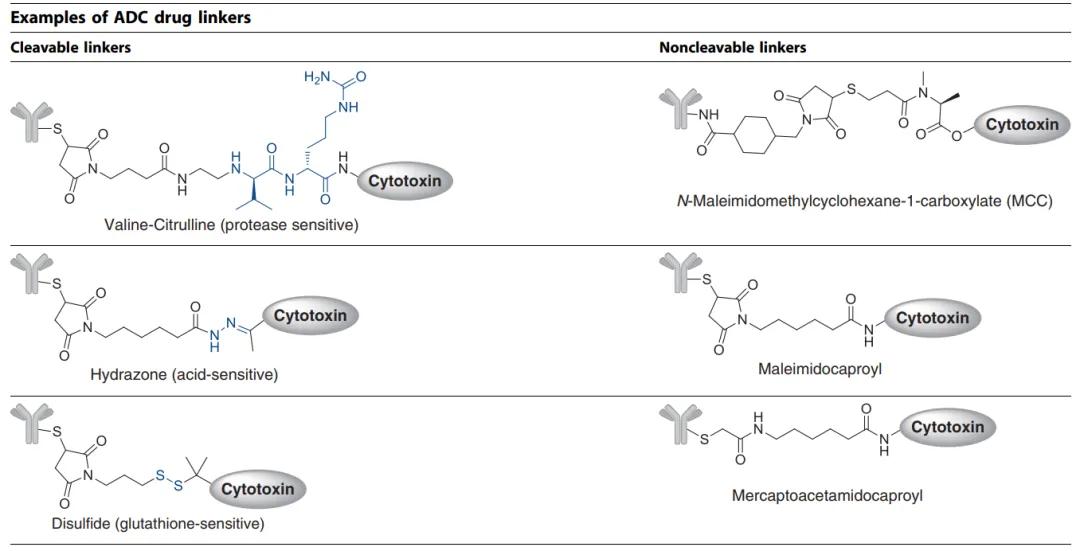
Common cleavable linkers include chemical reactive linkers, acid-labile linkers, reducible chain linkers, and enzyme-cleavable linkers. Non-cleavable linkers release cytotoxic payloads from antibody-drug conjugates within the lysosomal degradation process of the antibody-drug complex in the tumor environment, bypassing nonspecific dispersion of toxic agents. An advantage of non-cleavable linkers is the enhancement of stability in the bloodstream, thereby reducing off-target toxicity.
Mylotarg® employs a strategy utilizing acid-labile hydrazone linkers. Theoretically, hydrazone linkers should be stable in the physiological pH of the bloodstream and selectively hydrolyze under more acidic conditions post-internalization (with endosomal pH ranging from 5.0 to 6.5 and lysosomal pH from 4.5 to 5.0). However, reports indicate some instability of Mylotarg® linkers, leading to premature release of the payload in the bloodstream, potentially causing off-target toxicity. Benefiting from accumulated clinical knowledge in recent years, Pfizer reduced the dosage and modified the administration regimen of the drug, ultimately receiving re-approval from the FDA in 2017 [2,10].
Drug-Antibody Ratio (DAR)
The average number of payload molecules per antibody is typically referred to as the drug-antibody ratio (DAR). DAR determines the quantity of "payload" that can be delivered to tumor cells and can directly influence safety and efficacy. High drug loading often results in rapid plasma clearance, while ADCs with lower DAR exhibit diminished activity.
Conjugation Technologies
The successful design of ADCs in clinical settings relies not only on factors such as the potency and ratio of the payload, linker stability, and payload release but also on the choice of conjugation technologies.
Over the past decade, all FDA-approved ADCs have been composed of ADC mixtures with varying numbers of drugs attached to different positions on monoclonal antibodies. Currently, the industry has developed a range of novel conjugation strategies aimed at controlling the linkage position and quantity of payloads while maintaining structural integrity and homogeneity [5,9].
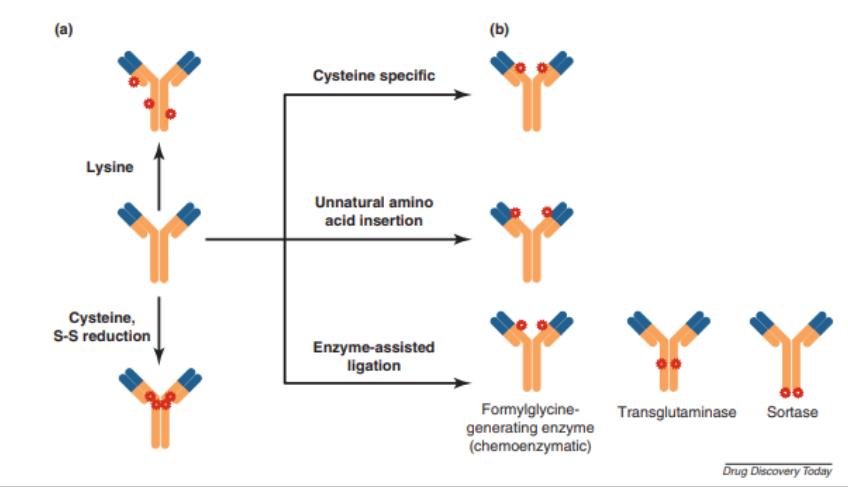
Stability of ADC Therapies[11]
The drug component concentration in marketed ADC Therapies formulations is often below 20 mg/mL, as their high selectivity and efficacy mitigate the need for high concentrations. Lower concentrations reduce the risk of aggregation, particularly for ADC molecules with hydrophobic payloads, which is an important advantage, as the solubility of ADCs may decrease compared to the parent antibodies. However, in clinical practice, where ADC drugs are often administered by infusion, the lower concentration of ADCs in infusion bags can pose challenges, especially considering the increased risk of drug loss due to adsorption onto plastic material surfaces.
For ADC products, liquid formulations may encounter stability issues, while lyophilized formulations can mitigate linker degradation during storage. Currently approved ADC drug formulations are predominantly lyophilized.
Another intriguing phenomenon is that some ADC formulations may be more photosensitive than traditional antibodies; for instance, Mylotarg®, Besponsa®, and Enhertu® are all packaged in amber vials.
Critical Quality Attributes (CQA) of ADC Therapies
According to ICH Q8 R2, critical quality attributes are defined and interpreted as "physical, chemical, biological, or microbiological properties or characteristics that should be within appropriate limits, ranges, or distributions to ensure the desired product quality (safety, efficacy)." Therefore, understanding the CQA of ADC drugs is of paramount importance in ADC drug development. Some common critical quality attributes of ADC drugs are listed in Table 3.
Table 3. Critical Quality Attributes of ADC Therapies (partial) [12]
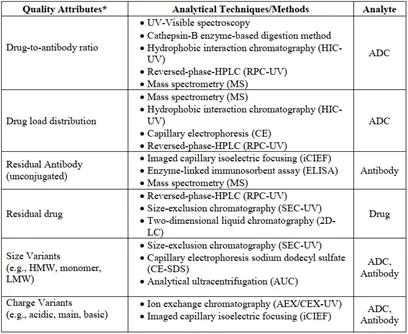
Analytical Techniques for ADC Therapies [12]
The application of analytical techniques pertinent to conventional antibody medicines may transfer to the analysis of ADCs. However, it should be emphasised that not all methodologies afford equivalent reliability and accuracy. For instance, if an ADC payload manifests significantly heterogeneous traits within samples, or absorbs UV light, or in the context of Size Exclusion Chromatography (SEC) where the ADC payload imparts hydrophobic features, it may necessitate mitigation of interactions with the chromatographic column through incorporating organic solvents in the mobile phase.
You may interested in
Below are outlined several quality parameters, unique to ADCs:
Determination of Drug-to-Antibody Ratio (DAR):
UV-visible spectroscopy serves as an uncomplicated and pragmatic technique to determine DAR, subject to certain conditions: 1) the existence of UV-visible chromophores within the payload; 2) distinct and separable absorbance peaks in the UV-visible spectra of the payload and antibody; 3) the assurance that the payload inclusion does not interfere with the absorption patterning of the antibody moiety, and reciprocally.
Hydrophobic Interaction Chromatography (HIC) capitalises on the abundance of hydrophobic payloads linked to antibodies for segregating conjugates. The antibodies in their least hydrophobic, unconjugated state are expelled initially from the column while conjugates with the highest DAR are last to be expelled. Alongside DAR, this method also provides insights into the ADC's level of conjugation, validating its ubiquity as a traditional chromatographic technique in the analysis of ADC medications.
Mass spectrometry serves as an invaluable approach for concretising ADC constructs by enabling the segregation, identification, and quantification of molecular entities. It also lends itself to DAR determination.
Determination of Payload Distribution
The overall payload distribution can be analyzed through mass spectrometry of intact molecules, and for ADCs with minimal heterogeneity generated through cysteine or other site-specific conjugation, hydrophobic interaction chromatography can also be utilized for characterization. For a more comprehensive analysis to determine the payload distribution at each conjugation site, techniques such as reduced mass spectrometric peptide mapping are required.
Reverse phase high-performance liquid chromatography (RPC) or capillary electrophoresis (CE), serving as orthogonal techniques, can provide information about the distribution of payloads on the light and heavy chains under reduced conditions, serving as complementary assays.
Measurement of Unconjugated Antibody
The level of unconjugated antibody is a critical parameter in process control as it directly impacts the efficacy of ADC therapeutics. Mass spectrometry serves as a common tool for quantifying unconjugated antibodies. Additionally, due to the inherent lipophilicity of payloads or linkers, the binding of hydrophobic payloads also alters the hydrophobicity of the protein molecules. Therefore, hydrophobic interaction chromatography can also be utilized to determine the levels of unconjugated antibodies in ADC samples.
Given that the binding of payloads to antibodies can greatly alter the surface and overall charge distribution, techniques such as whole-column imaging capillary isoelectric focusing (icIEF) are also viable analytical tools. Furthermore, other methods such as reverse phase high-performance liquid chromatography, capillary electrophoresis, enzyme-linked immunosorbent assay (ELISA), among others, can be explored.
It is worth noting that mass spectrometry analysis serves as a powerful tool for characterizing ADC structures. It plays an indispensable role in ADC process development and quality analysis. The common applications of mass spectrometry in ADC quality analysis are outlined in detail in Figure 10.
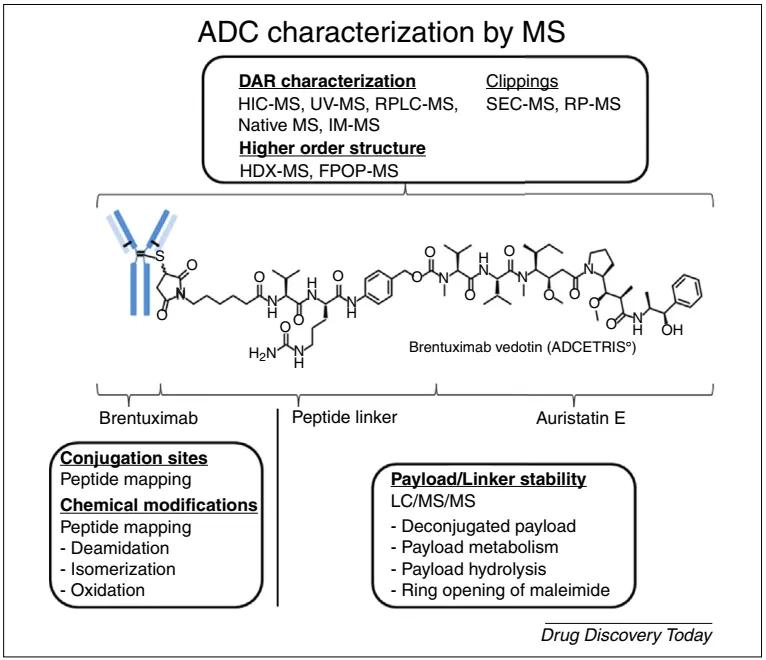
Development and Manufacturing of ADC Therapies [14]
Process Development
Compared to any other category of active pharmaceutical ingredients (APIs), the quality of ADC drugs is more constrained by the manufacturing process. A robust production process is indispensable for the reproducible manufacture of a subset of compounds with heterogeneous compositions. To ensure process consistency, thorough research and control of numerous parameters in the conjugation process are essential. The selection of appropriate processing, storage, and handling conditions during production should be based on studies of the physical and chemical stability of intermediates and ADCs.
Refined process development also demands experienced and well-trained experimental personnel, along with suitable laboratory equipment.
In order to conduct more experiments with minimal materials, micro-scale reactors capable of coupling experiments at the milligram level while maintaining precise temperature control have proven highly useful. Such reaction models have been demonstrated to simulate the performance of reactions on scales of up to several hundred liters. However, for purification processes, kilogram-scale operations are required to evaluate tangential flow filtration (TFF) conditions.
Scale-Up Manufacturing
The most significant challenge in ADC drug production may lie in the design, construction, and operation of biomanufacturing environments that allow for the safe handling of potent cytotoxic drugs and their contaminants (e.g., materials containing toxic substances, waste disposal). Therefore, emphasis should be placed on personnel training to ensure the correct use of equipment and adherence to safety protocols. Additionally, a personal protective equipment plan should be developed to cover residual risks that cannot be eliminated by engineering controls and contingency plans for the leakage of toxic compounds.
Equipment cleaning is another critical activity when manufacturing potent cytotoxic drugs. Disposable technologies can reduce the costs of cleaning operations, but the materials are often expensive, and the provision of leachables and extractables data may be required. Therefore, glass or stainless steel containers are typically preferred for conjugation reactions.
The validation of cleaning procedures for ADC production lines requires highly sensitive detection methods. Traditional methods based on total organic carbon (TOC) and high-performance liquid chromatography (HPLC) may not provide sufficient sensitivity for detecting residual cytotoxins, necessitating the use of validated ELISA or LC-MS analytical methods.
Prospects and Challenges of ADC Therapies
Despite the complexity of ADC design, the prospects of this therapeutic approach have garnered significant interest in recent decades. The robust ongoing clinical pipelines and the continuous approval of ADC drugs serve as prime examples of promising prospects[9]. Beyond their widespread application in oncology, the concept of ADCs also holds potential in other therapeutic areas, such as treating methicillin-resistant Staphylococcus aureus infections, vancomycin-resistant intracellular Staphylococcus aureus infections, or inflammation[1].
The current approach for selecting lead ADC candidates in clinical trials typically relies on systematic in vitro evaluations of various antibodies, linkers, and cytotoxic payloads, although the adequacy of in vitro models to predict drug responses remains to be fully observed. Early in vivo studies may prove crucial before further understanding ADCs [9].
One of the primary challenges of ADC drugs is off-target toxicities, resulting from the premature release of cytotoxic small molecules into the bloodstream. Clinically reported off-target toxicities include hepatotoxicity, thrombocytopenia, peripheral neuropathy, neutropenia, anemia, and ocular toxicity [9, 10, 15]. Additionally, the complex mechanisms of potential drug resistance with ADCs need further clarification. Identifying resistance mechanisms to ADCs from other administered compounds in the context of treatment combinations poses significant challenges [9, 10, 15].
Despite the numerous challenges in the design, process, and development of ADC products and clinical practice, further clinical trials and basic research on existing ADCs pave the way for addressing issues related to tumor markers, antibodies, cytotoxic payloads, and conjugation strategies. The future of ADC Therapies is promising.
References
- Antibody–Drug Conjugates—A Tutorial Review. Molecules 2021, 26, 2943
- Antibody-Drug Conjugate-Based Therapeutics: State of the Science. JNCI J Natl Cancer Inst (2019) 111(6): djz035
- Antibody–Drug Conjugates for Cancer Treatment. Annu. Rev. Med. 2018. 69:191–207
- The Chemistry Behind ADCs. Pharmaceuticals 2021, 14(5), 442
- Antibody–Drug Conjugates—A Tutorial Review. Molecules 2021, 26, 2943
- Antibody-Drug Conjugates: Possibilities and Challenges. Avicenna Journal of Medical Biotechnology, Vol. 11, No. 1, January-March 2019
- Introduction to Antibody-Drug Conjugates. Antibodies 2021, 10, 42
- An Insight into FDA Approved Antibody-Drug Conjugates for Cancer Therapy. Molecules 2021, 26, 5847
- Antibody–drug conjugates: current status and future directions. Drug Discovery Today, Volume 00, Number 00, Dec 2013
- Antibody–Drug Conjugates: The Last Decade. Pharmaceuticals 2020, 13, 245
- Antibody-drug conjugates- stability and formulation. European Journal of Pharmaceutics and Biopharmaceutics 139 (2019) 168–176
- Challenges and new frontiers in analytical characterization of antibody-drug conjugates. MAbs. 2018 Feb/Mar;10(2):222-243
- Characterization of antibody–drug conjugates by mass spectrometry: advances and future trends. Drug Discovery Today, Volume 00, Number 00, April 2016
- Chapter 29: Challenges in the Development and Manufacturing of Antibody–Drug Conjugates,489-497
- Advances and Limitations of Antibody Drug Conjugates for Cancer. Biomedicines 2021, 9, 872