Overview of Host Cell Proteins (HCPs)
Sources of HCPs
Host cell proteins (HCPs) are proteinaceous components derived from the host cells used in production cell lines. They encompass both structural and secreted proteins originating from the host cells. The primary source of HCPs is cell lysis, and their levels correlate with cell density and viability at the time of harvest. Typically, under identical conditions of viable cell density (VCD), a lower viability at harvest results in higher HCP levels. Consequently, during upstream cell culture, it is standard practice to maintain cell viability at harvest above 70%.
Impact of HCPs
HCPs, as process-related impurities, are present at low concentrations in recombinant biopharmaceutical products. Despite their typically low levels, HCPs can adversely affect the properties of the drug. For patients, HCPs are considered xenogenic proteins, which may possess immunogenic properties. Therefore, even minute quantities of HCPs can potentially trigger adverse immune reactions. Additionally, HCPs may include proteolytic enzymes, which can fragment the target protein, thereby reducing the overall yield of the product. Besides degradation and fragmentation of the target protein, HCPs can also induce protein aggregation and other detrimental effects.
Detection of HCPs
The most commonly employed method for detecting HCPs in the production of therapeutic proteins is the enzyme-linked immunosorbent assay (ELISA). This technique utilizes polyclonal antibodies to quantitatively analyze the total abundance of HCPs. However, ELISA generally cannot provide rapid quantification of individual HCP components and may fail to detect weakly immunogenic or non-immunogenic HCPs. Complementary analytical techniques, including 1D/2D-polyacrylamide gel electrophoresis (PAGE) and mass spectrometry-based methods, have been developed for HCP monitoring. Among these, liquid chromatography-tandem mass spectrometry (LC-MS/MS) can simultaneously identify and quantify HCP impurities and serves as a major complementary method to ELISA.
Control of HCPs
Upstream Control
To mitigate the presence of HCPs, it is critical to control the viability of cells at harvest, with higher viability being preferable.
Downstream Clearance
Downstream processes for HCP clearance include depth filtration, affinity chromatography, anion exchange chromatography, and cation exchange chromatography.
Establishment of Quality Standards
Due to the potential adverse effects of HCPs on human safety, it is generally accepted to set the quality standard for residual HCPs in the drug substance at a level not exceeding 0.01% of the total protein content.
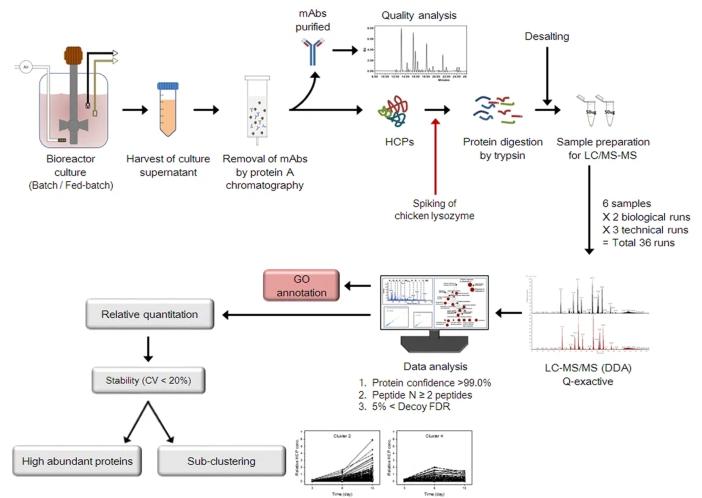
Removal of HCPs by Depth Filtration
Introduction to Depth Filtration
Depth filtration is a commonly employed method for pre-filtration processes, including clarification of fermentation broths, removal of cell debris and DNA, and pre-treatment of blood products and other biological substances. The mechanism underlying depth filtration primarily relies on size exclusion and adsorption principles.
Size Exclusion: The filtration process involves the retention of viruses, which are larger than the pore size of the filter membrane, while allowing the product to pass through.
Adsorption Mechanism: Viruses smaller than the pore size can be captured via adsorption through electrostatic forces, van der Waals interactions, and hydrogen bonding.
HCP Removal by Depth Filtration
In addition to the mechanical sieving of cell debris and large particulates, depth filters with positive charge characteristics can adsorb soluble submicron particles. Consequently, depth filtration is a key step in the comprehensive removal of HCPs. It is important to note that the effectiveness of HCP removal is significantly influenced by the characteristics of the feed, such as the nature of the fermentation broth or the centrifuged harvest fluid. As the filtration system approaches saturation, the efficacy of HCP removal decreases, leading to breakthrough as the load increases.
Multistage depth filtration, involving a series of depth filters, provides enhanced HCP removal efficiency. During the selection and scale-up evaluation of filters, the capacity of depth filters to remove HCPs is often an overlooked parameter.
Removal of HCPs via Affinity Chromatography
Introduction to Affinity Chromatography
Affinity chromatography constitutes an essential methodology for the purification of monoclonal antibodies (mAbs), with Protein A recognized as the predominant affinity ligand in this context. The Protein A chromatography step is typically the initial unit operation within the purification cascade. Protein A, a cell wall protein derived from Staphylococcus aureus with an approximate molecular weight of 42 kDa, exhibits specific affinity for the Fc region of various immunoglobulins.
Protein A encompasses five distinct domains that bind with high specificity to the Fc fragment of immunoglobulin G (IgG) molecules. This protein is immobilized on an agarose matrix, thereby enabling the selective adsorption of antibody molecules while permitting non-target proteins to be eluted. The immobilization enhances the specificity and efficiency of the purification process. Each Protein A molecule has the capacity to bind at least two IgG molecules. Moreover, Protein A demonstrates binding affinity to other immunoglobulin classes, including immunoglobulin A (IgA) and immunoglobulin M (IgM), from specific species.
This structured and selective interaction underscores the significance of Protein A in the affinity chromatography of mAbs, enabling high-purity isolation crucial for downstream applications and therapeutic use.
Impurity Removal: During the affinity chromatography process, process-related impurities, including HCPs, DNA, and small molecules, are removed in the flow-through or wash steps. The bound monoclonal antibodies are subsequently eluted using a low pH buffer, followed by a low pH inactivation step. Although Protein A chromatography has demonstrated virus removal capability, this function is generally not claimed as part of the standard antibody purification process.
HCP Removal via Affinity Chromatography
Due to the high affinity of Protein A for antibodies, stringent conditions are required to elute the bound antibodies. Consequently, affinity chromatography represents one of the most effective unit operations for the removal of HCPs in downstream processing. This step alone can eliminate more than 90% of HCPs from clarified harvest fluids, typically reducing the host cell protein content from 10^5 ppm to a range of 2000–10000 ppm. However, the level of HCPs in the affinity eluate can vary significantly depending on the nature of the starting material.
There are two primary concerns regarding HCP removal during Protein A affinity capture chromatography: First, HCPs can nonspecifically bind to the Protein A resin, particularly to resins with organic polymer backbones. Second, HCPs can nonspecifically associate with the monoclonal antibody itself. In general, higher concentrations of additives and higher pH wash buffers (e.g., pH 9.0) are more effective in disrupting mAb-HCP interactions while maintaining good product recovery. The use of these wash additives in combination can enhance the removal efficiency.
Removal of HCPs via Anion Exchange Chromatography
Introduction to Anion Exchange Chromatography
Anion exchange chromatography (AEX) is widely employed in the purification of mAbs, particularly when the isoelectric point (pI) of the mAb is greater than 7. Under neutral pH conditions, most proteins carry a net positive charge and thus exhibit a flow-through behavior on an anion exchange medium. This technique is highly effective for the removal of impurity proteins, aggregates, endotoxins, and most notably, viruses, thereby enhancing drug safety. Many viruses, being negatively charged at pH values above 7, are captured by positively charged quaternary ammonium groups on the chromatography resin, facilitating their removal. In the case of bispecific antibodies, which often have a lower pI (sometimes as low as 5), an anion binding and elution mode can be utilized for purification. It is crucial to maintain a low sodium chloride concentration in the elution buffer to prevent the co-elution of impurities, viruses, and endotoxins, which would otherwise compromise impurity removal. Composite resins such as MaXtar MMA or MaXtar MMC can also be considered for enhanced purification performance.
HCP Removal via Anion Exchange Chromatography
In AEX, the flow-through mode is typically employed, where samples are applied at a pH below the pI of the target protein and under low conductivity conditions. In this mode, negatively charged impurities bind to the resin while the target protein flows through. Although the flow-through mode can effectively remove HCPs and other impurities, enhanced impurity removal can be achieved by operating the column under a weak adsorption mode. This mode involves selecting appropriate pH and conductivity conditions to weakly retain the target protein on the resin (with a product partition coefficient, k, ranging from 0.1 to 20, preferably between 1 and 3). Under these conditions, impurities bind more strongly to the resin than the target protein, facilitating greater HCP removal. The weak adsorption mode can be further optimized by incorporating intermediate washes or increasing the load to mitigate any reduction in product recovery. An optimized weak adsorption mode can achieve up to a 4-log reduction value (LRV) in HCPs, a significant improvement compared to the less than 1 LRV achieved in flow-through mode.
HCP Removal in Other Chromatographic Steps
Additional chromatographic steps in the polishing phase, including cation exchange chromatography (CEX), hydrophobic interaction chromatography (HIC), and mixed-mode chromatography, play crucial roles in HCP removal. Among these, mixed-mode resins are particularly notable due to their ligands' ability to mediate multiple types of interactions. These resins are increasingly employed for HCP removal and impurity clearance.
Studies have demonstrated that during or after virus inactivation, caprylic acid can be effectively used to precipitate HCPs. Findings indicate that 1% caprylic acid is sufficient for low-pH inactivation, with an optimal precipitation pH range of 5.0 to 5.4. Various strategies can be applied at different stages of the downstream process.
While understanding the properties of HCPs can guide the design of further purification strategies, developing a tailored purification method for each individual HCP is impractical. However, identifying and targeting common HCPs for removal can significantly reduce the overall HCP levels in many products.
Summary of Strategies for HCP Clearance
HCPs, originating from production cell lines, represent substantial obstacles in the biopharmaceutical manufacturing process as they possess immunogenic potential and can lead to the degradation of therapeutic proteins. Effective strategies for HCP clearance encompass both upstream and downstream processes with an emphasis on maintaining high cell viability at harvest to minimize initial HCP concentrations.
Downstream approaches for HCP clearance incorporate depth filtration, affinity chromatography, and AEX, among other chromatographic techniques. Depth filtration aids in the removal of cell debris and soluble HCPs through mechanisms of size exclusion and adsorption. Affinity chromatography, particularly Protein A, demonstrates high specificity for immunoglobulins and can significantly reduce HCP concentrations via stringent elution conditions. AEX in flow-through mode effectively binds negatively charged impurities, thereby enhancing HCP removal efficiency.
Subsequent chromatographic procedures, including CEX, HIC, and mixed-mode chromatography, contribute to a thorough HCP clearance. Mixed-mode resins, owing to their multifaceted interaction capabilities, are particularly efficacious in decreasing HCP levels. The optimization of processes, involving tailored pH and conductivity conditions as well as intermediate wash steps, further augments impurity removal.
Overall, the comprehension and implementation of effective HCP removal strategies are paramount for ensuring the safety and efficacy of biopharmaceutical products. The integration of multiple purification techniques facilitates the systematic reduction of HCPs, thereby conforming to stringent quality standards and regulatory requirements.
References
- Park, J., Jin, J., Lim, M. et al. Proteomic Analysis of Host Cell Protein Dynamics in the Culture Supernatants of Antibody-Producing CHO Cells. Sci Rep 7, 44246 (2017).
- Kornecki, M.; Mestmäcker, F.; Zobel-Roos, S.; Heikaus de Figueiredo, L.; Schlüter, H.; Strube, J. Host Cell Proteins in Biologics Manufacturing: The Good, the Bad, and the Ugly. Antibodies 2017, 6, 13.