Currently, recombinant monoclonal antibodies are predominantly produced using mammalian cell expression systems. Throughout the production, storage, and transport stages, issues like product aggregation, degradation, and various post-translational modifications typically occur. These issues can lead to heterogeneities in the product's molecular size, charge, glycosylation, among other properties, along with the generation of corresponding variants. These variants can materialize at any point throughout the product's life cycle. Some data indicate that heterogeneity strictly attributable to post-translational modifications, such as N-terminal glutamic acid cyclization, C-terminal lysine removal, deamidation, oxidation, sialic acid modification, etc., can reach up to 2.85 × 108.
The variants, induced by diverse influencing factors, can change the charge attributes of the recombinant protein product by altering the quantity or structure of charged groups. Consequently, this can generate different charge heterogeneity within the same protein product.
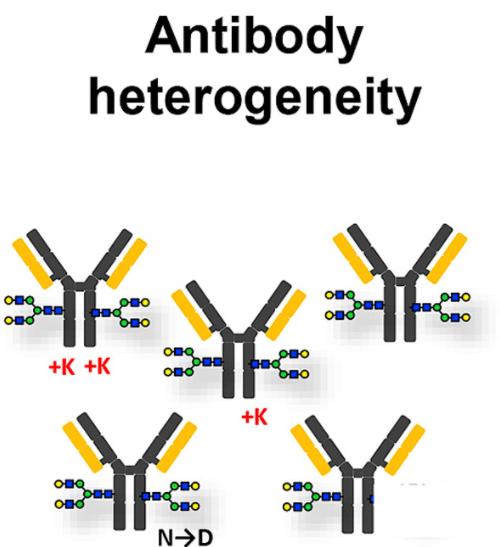
Select services
Charge Heterogeneity in Recombinant Monoclonal Antibodies
Heterogeneity of recombinant proteins, modified proteins characterized by varied electrical charge attributes, can be purified using charge separation methodologies, generating divergent peaks, most notably acidic, main and basic peaks. The alterations in the number or the structure of the charge groups, induced either directly or indirectly through conformational changes prompted by various modifications such as glycosylation, deamidation, isomerization, oxidation, cleavage and aggregation, can trigger fluctuations in the surface charge of the proteins, culminating in charge heterogeneity in the recombinant proteins and antibodies.
The charge heterogeneity of recombinant antibodies exerts significant influence on its efficacy and safety. Numerous factors contribute to the formation of charge heterogeneity in antibody proteins. A comprehensive analysis of these molecular bases and contributing factors can provide precise control and adjustment during the development of antibody drugs.
Of note is that acidic heterogeneity might exert more adverse impact on the drug activity of antibodies, especially the modifications in complementary determining region. For instance, the HER2 affinity of trastuzumab's acidic charge heterogeneity is notably reduced, leading to a weakened anti-proliferative activity. As such, in the development of antibody drugs, reducing the proportion of acidic charge heterogeneity is often considered a higher priority over reducing the basic charge heterogeneity.
In order to manage the impact and regulation of antibody charge variants, we need to deeply understand and master the relevant foundational knowledge to ensure the safety and efficacy of antibody drugs.
Source of Charge Heterogeneity
The primary sources driving the formation of basic charge variants in antibodies comprise: the removal of C-terminal lysine, the formation of N-terminal pyroglutamic acid, isomerization of aspartic acid, and amidation of proline. In contrast, acidic charge variants principally stem from deamidation of asparagine, glycation of lysine, mismatched disulfide bonds, trisulfide bond formation, and the sialic acid elements introduced during the glycosylation process.
Terminal Lysine Modification
The modification of the C-terminal of the heavy chain in an antibody molecule encompasses the removal of C-terminal lysine and the amidation of proline. Studies indicate that for antibody molecules like IgG1, IgG2, and IgG3, the heavy chain's C-terminus invariably ends with a Pro-Gly-Lys amino acid sequence. During this process, the terminal lysine is cleaved by intracellular and extracellular carboxypeptidases during the cell culture stage, exposing the penultimate amino acid glycine, a step referred to as the C-terminal lysine cleavage. However, in some instances, lysine residues may not be completely removed, resulting in the production of antibodies containing structural variants with unclipped C-terminal lysine. These variants are known as lysine variants. Given that lysine is a basic amino acid, its incomplete removal can elevate the isolectric point of the protein, thus classifying lysine variants as basic charge variants.
Deamidation and isomerization
Deamidation and isomerization play essential roles in the process of asparagine (Asn) degradation during the production and storage of antibodies, initiating the formation of acidic charge variants. In a neutral or alkaline pH environment, the process of Asn deamidation involves the formation of a five-membered ring intermediate, the succinimide. Specifically, the nitrogen atom on the peptide bond undergoes deprotonation and subsequently attacks the carbonyl carbon on the side chain, leading to the loss of an amine to form succinimide, which is an irreversible process. The produced succinimide is unstable and further hydrolyzes to aspartic acid (Asp) and isoAsp in a reversible manner. Thus, aspartic acid residues in the antibody molecule may spontaneously dehydrate to form succinimide, which can further hydrolyze to Asp and isoAsp. This process is referred to as the Asp isomerization. At acidic pH values, Asn deamidation is primarily achieved through the direct hydrolysis of the side-chain amine, producing only Asp. Given that the isoelectric point of Asn is higher than all its potential products, the deamidation of Asn can lead to the formation of acidic charge variants in antibodies, while the isomerization of Asp can trigger the formation of basic variants.
Sialic Acid
Sialic acid, a critical monosaccharide derivative that constitutes the termini of glycosylated antibody chains, is impacted by various factors, including the type and source of the antibody. Typically, antibodies in human serum contain approximately 10% sialic acid. However, it's notable that recombinant monoclonal antibodies expressed through CHO cells generally possess less than 2% sialic acid, while fusion proteins using the same expression system demonstrate higher levels of sialic acid. Additionally, sialic acid in human antibodies is primarily present as N-acetylneuraminic acid (NANA). However, in murine cell lines (such as NS0, SP20) and recombinant proteins expressed in CHO cells, sialic acid is found in the form of both NANA and N-glycolylneuraminic acid (NGNA).
Because sialic acid possesses acidic properties, its presence contributes to the formation of acidic charge variants in antibodies. Importantly, sialic acid significantly prolongs the half-life of antibody drugs in vivo. If the terminal glycan of a glycoprotein fails to be sialylated during modification, the glycoprotein will be recognized and bound by asialoglycoprotein receptors (ASGPRs) in human liver and rapidly cleared from the system.
Oxidation
The amino acid Methionine, also known as Met, is the most susceptible to oxidation within antibodies. In environments with a high oxidative stress, Met undergoes an oxidation process, transforming into Methionine sulfoxide. Although this process does not involve a net charge alteration, it often triggers changes in the spatial charge distribution of the antibody, consequently leading to the formation of charge variants. Studies indicate that oxidation of Met could result in the generation of both acidic or basic charge variants.
The easiness of Met oxidation is closely associated with the solvent environment it is located in. Research shows that Met252 and Met428 are not only the Met residues in antibodies most prone to oxidation, but their oxidation also impedes the binding between antibodies and neonatal Fc receptor (FcRn). This hindrance makes antibodies more susceptible to intracellular lysosomal degradation and thereby leads to the shortening of the half-life of antibody drugs in the body.
Moreover, oxidation of Met decreases the antigen-binding capacity and thermal stability of antibody drugs. Research conducted by Kroon unveiled that when Met in the heavy chain CDR region of the OKT3 monoclonal antibody is oxidized, a significant decrease in the antigen-binding capacity of the monoclonal antibody is observed. Through a semi-preparative AEX method, Teshima et al. succeeded in the separation of charge variants caused by Met oxidation. Using differential scanning calorimetry (DSC) for the evaluation of the impact of Met oxidation on antibody thermal stability, their findings show that oxidation of Met in the CDR2 region significantly reduces the thermal stability of the antibody. Therefore, Met oxidation is generally scrutinized as a critical quality attribute (CQA) of antibody drugs.
Glycosylation
Glycosylation refers to an intrinsic biological process where proteins or lipids are conjugated with glycan chains under enzymatic activity, representing a pivotal post-translational modification occurring within biological entities. Glycosylation unfolds within the endoplasmic reticulum and Golgi apparatus, mediated by specific glycosyltransferases, and exhibits a definitive site specificity. Unlike protein synthesis, the glycosylation process in antibodies lacks template direction and its magnitude and type of glycosylation are susceptible to the cell type and cultivation conditions. Consequently, the analysis of glycosylation sites and glycan composition entails a highly intricate process.
In monoclonal antibodies produced by humans and CHO cells, a prevalent glycosylation site is Asn297, found in the conservatively sequenced N-linked glycosylation site of the antibody's Fc segment. The glycosylation structure at this site entails two N-linked biantennary or multi-antennary complex-type oligosaccharides. Diverse monosaccharide transferases append fucose, galactose, mannose or sialic acid at the termini of these oligosaccharides, thus bestowing the antibody with substantial heterogeneity.
Notably, polysaccharide chains usually display neutrality. However, when the terminal galactose residues on the glycan chain increase and are accompanied by negatively charged sialic acid, a shift in charge occurs. Variations in the degree of sialylation often contribute to the common cause of charge heterogeneity in antibodies. Nevertheless, the level of sialic acid modification remains low in antibodies produced in humans and CHO cells.
You may interested in
Disulfide bonds
Disulfide bonds serve as crucial connectors between subunits in protein molecules, playing a pivotal role in maintaining protein tertiary structure and biological activity. Within monoclonal antibodies, disulfide bonds take two forms: interchain and intrachain, with differing formation patterns among various antibody isotypes. These accurate bond formations primarily occur in the endoplasmic reticulum of cells. However, due to a myriad of systemic factors, these bonds can undergo an array of erroneous chemical modifications, including mismatches, reductions, formation of trisulfide bonds, thioether bond formation, sulfonation, amidation, citrullination, and cysteinylation.
Worth noting is that the reduction of interchain disulfide bonds could lead to the generation of reduced antibody fragments, such as HHL antibodies (missing one light chain) and HH antibodies (missing two light chains). These chemical modifications often induce changes in the antibody's spatial structure and charge distribution, resulting in the generation of charge variants. The types of chemical modifications associated with disulfide bonds within an antibody molecule are tremendous, and the impacts of these modifications on the efficacy and safety of the drug differ considerably.
Research indicates that mismatches in disulfide bonds, cysteinylation, and reduction of disulfide bonds typically result in a decrease in drug affinity, biological activity, and thermal stability. The formation of trisulfide bonds, on the other hand, does not show significant impacts on the effectiveness or safety of antibody drugs. Moreover, the influence of thioether bond formation, sulfonation, amidation, and citrullination of disulfide bonds on the functionality of antibody drugs remains ambiguous, warranting further comprehensive investigation.
Causes of Charge Heterogeneity
The origins of charge heterogeneity in biotherapeutic recombinant proteins are decidedly multifaceted and bound up in complex functional mechanisms. A profound understanding of these mechanisms is crucial to effectively regulate the generation of charge heterogeneity during production. Current studies suggest charge heterogeneity may emerge at multiple stages of the production processes, encompassing cell line development, fermentation, and purification stages. Environmental factors such as elevated temperatures, intense light, or exposure to certain chemicals can also potentially trigger charge heterogeneity.
The causative factors tie in with a broad spectrum of types of antibody modifications, which includes both enzymatic and non-enzymatic reactions. These range from disulphide bond formation, glycosylation, isoAsp formation, C-terminal Lys processing, deamidation, oxidation, glycation, isomerization to peptide bond cleavage.
The formation of charge isoforms strongly correlates with the primary and tertiary protein structures. For instance, in the study by Yang et al., two critical degradation sites were identified in Trstuzumab monoclonal antibodies, specifically at LC-Asn 30 and HC-Asp 102. When these sites were replaced by specific amino acids, there was a marked regulation of the degradation rate of the monoclonal antibodies and the generation of charge isoforms. The study further underlined that deamidation rates of Asn in antibodies were closely related to storage conditions such as temperature, pH, and ionic strength. The oxidation rate of Met showed a close association with the redox condition of the storage environment and the concentration of metal ions. To ensure minimal generation of charge isoforms in biotherapeutic recombinant proteins during storage, a meticulous optimization and development of formulation and storage conditions are imperative.
Analysis of Charge Heterogeneity
Imaged capillary isoelectric focusing (icIEF) is an advanced microseparation technique that exploits the isoelectric point (pI) properties of proteins, serving as an effective means to reveal protein charge heterogeneity. Its working principle involves the application of a direct voltage at both ends of the capillary, triggering the formation of a specific pH gradient by amphoteric electrolyte carriers within. In this environment, depending on the charges they carry, proteins migrate towards the anode or cathode. Upon reaching a specific pH value where their net charge is zero (i.e., the isoelectric point pI), their movement ceases. Ultimately, proteins focus into narrow zones within the capillary, thereby achieving effective separation.
Select services
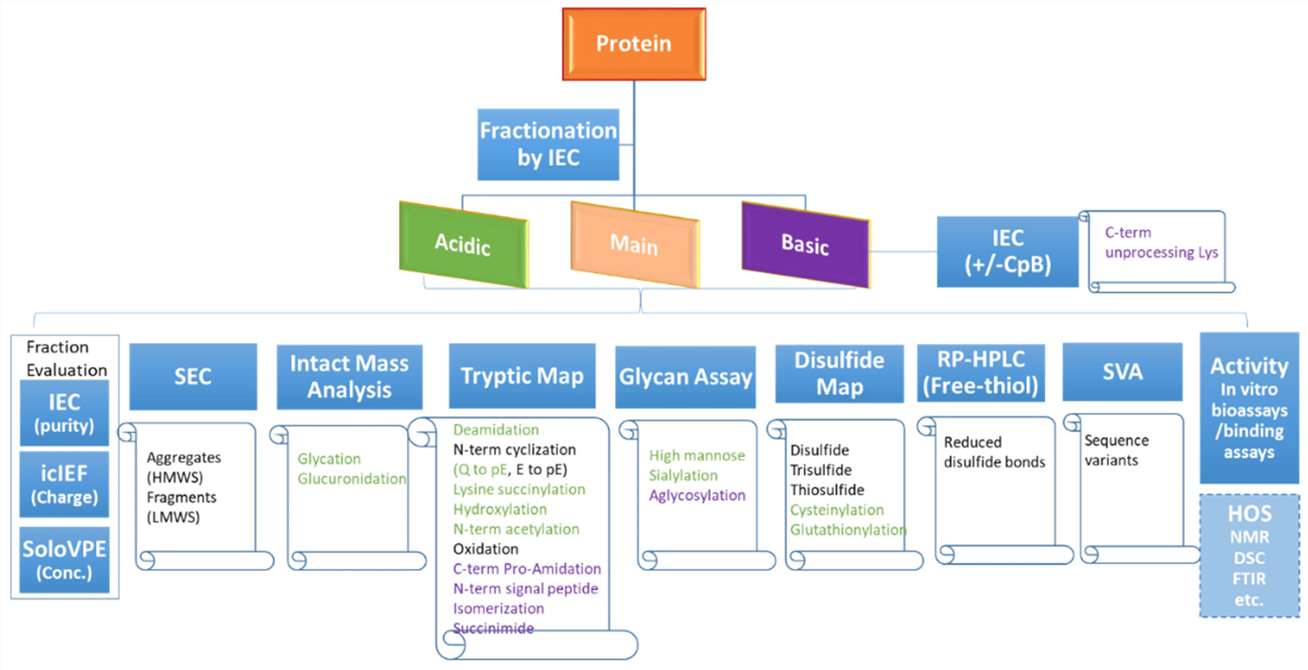
Regulation of Charge Heterogeneity
There is ample evidence suggesting that the proportion of acidic charge variants in monoclonal antibodies can be reduced effectively by decreasing the cultivation temperature, simultaneously elevating the content of the main peak component. The pH value represents a crucial determinant during this cultivation process, prominently impacting the dispersion of antibody charge and the content of acidic charge variants. Further reduction in the proportion of acidic charge variants of monoclonal antibodies can be achieved through lowering the pH value of the cultivation system.
Moreover, light exposure has a notable influence on the charge heterogeneity of monoclonal antibodies, especially within small-scale reactors, where it often presents as the primary factor contributing to the elevation of acidic charge variant content. Subsequent analysis posits that light exposure may alter the three-dimensional arrangement and charge distribution of monoclonal antibodies, thereby affecting their charge heterogeneity.
Within the cultivation medium, metal ions, including copper ions and zinc ions, also shape the charge heterogeneity of monoclonal antibodies. Copper ions are capable of inhibiting the activity of intra- and extracellular alkaline carboxypeptidase, while zinc ions can activate the same enzyme, thus affecting the cleavage of C-terminal lysine in the heavy chain of the antibody and the amount of lysine variants in the end product.
Additives in the cultivation medium, such as sodium butyrate, dexamethasone, and hydrocortisone, also impact the charge heterogeneity of antibody molecules. With its function of inhibiting histone deacetylase activity, sodium butyrate is commonly employed to stimulate product expression. Recent research suggests that sodium butyrate also plays a vital role in the regulation of antibody glycosylation and charge heterogeneity, among other quality aspects.
References
- Josef Vlasak a, Marie C. Bussat b, Shiyi Wang a, Elsa Wagner-Rousset b, Mark Schaefer a,Christine Klinguer-Hamour b, Marc Kirchmeier a, Nathalie Corvaïa b, Roxana Ionescu a,*, Alain Beck b,*:Identification and characterization of asparagine deamidation in the light chainCDR1 of a humanized IgG1 antibody. Analytical Biochemistry 392 (2009) 145–154
- Hong L. Yao1,2 & Louis P. Conway1 & Mao M. Wang1 & Kun Huang1 & Li Liu1 &Josef Voglmeir 1:Quantification of sialic acids in red meat by UPLC-FLDusing indoxylsialosides as internal standards. Glycoconj J
- J. Vlasak* and R. Ionescu: Heterogeneity of Monoclonal Antibodies Revealed by Charge-SensitiveMethods. Current Pharmaceutical Biotechnology, 2008, 9, 468-481
- HONGCHENG LIU,' GEORGEEN GAZA-BULSECO,' DINESH FALDU, CHRIS CHUMSAE JOANNE SUN:Heterogeneity of Monoclonal AntibodiesReceived 14 May 2007: revised 2 July 2007: accepted 31 July 2007 Wilev InterScience