Charged particles, such as ampholytic proteins and nucleic acids, exhibit a unique characteristic: they attain equilibrium of positive and negative charges carried by the particles under specific pH conditions. In this state, the particles do not migrate in an electric field, a condition known as the isoelectric state. The pH value at this state is defined as the isoelectric point of the charged particle. In essence, the isoelectric point refers to the pH value at which the surface charge of the particle is zero. Upon reaching the isoelectric point, various physical properties of ampholytic electrolytes, such as solubility, viscosity, and osmotic pressure, minimize, a phenomenon particularly evident in protein solutions.
pH
The term "pH" serves as a descriptor for the hydrogen ion active concentration in a solution or, alternately, the degree of acidity or alkalinity therein. The formal definition of pH can be derived from the equation: pH = -log [H⁺], where the bracketed [H⁺] symbolizes the activity of hydronium ions, denoted [H₃O⁺], and typically expressed in molarity (mol·L⁻¹).
Under the presumed conditions of thermodynamic normality, the pH values convey distinct meanings: a pH of 7 is synonymous with neutrality, while a pH falling below 7 is indicative of acidity, and a value above 7 is suggestive of alkalinity. The corresponding acidity or alkalinity of an aqueous solution can further be elucidated via pOH, the negative logarithm representing hydroxide ion concentration. Through the process of water autoionization equilibrium, one can verify that at 298K, the sum of pH and pOH values will invariably equate to 14.
A pH value that sits below 7 reflects a preponderance of H⁺ ions over OH⁻ ions, and thus corresponds to a higher degree of acidity in the solution. On the other hand, a pH that registers above 7 is indicative of a larger concentration of OH⁻ ions relative to H⁺ and thus suggests heightened alkalinity. Hence, as the pH value diminishes, the solution's acidity escalates in direct relation, and as the pH value rises, the solution's alkalinity correspondingly intensifies.
However, in cases involving non-aqueous solutions or circumstances that deviate from the standard temperature and pressure, a pH of 7 may not necessarily epitomize neutrality. Delimiting the neutral pH value under these specific conditions necessitates the computation of the solvent's ionization constants.
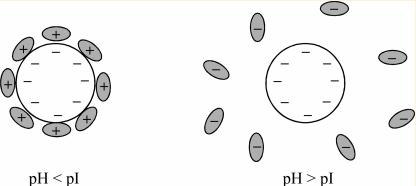
Isoelectric Point
The charge carried by amphoteric ions varies with the pH of the solution. The isoelectric point of a solution is reached when the positive and negative charges of amphoteric ions are equal.
When the pH of the external solution exceeds the pI value of amphoteric ions, these ions release protons, resulting in a negative charge. Conversely, when the pH of the external solution is less than the pI value of amphoteric ions, these ions protonate, acquiring a positive charge. At the isoelectric point, the solubility of amphoteric ions in the solution is minimized.
pKa and pKb
Within a water-based solution, acids such as HA participate in a dissociation process, represented by the equation HA + H₂O ⇆ A⁻ + H₃O⁺. This relates to the notion of the acid dissociation constant, Ka, which is expressed mathematically as: Ka = [H₃O⁺] × [A⁻] / [HA]. However, in light of the broad range of Ka values, chemists often utilize the term pKa as a measure of acidity, defined as pKa = -log(Ka).
The relationship between pKa and Ka is inverse; as the Ka value increases, signaling stronger acidity, the corresponding pKa value decreases. On the other hand, higher pKa values suggest weaker acidity. It is important to note that the magnitude of the pKa is influenced by the structure of the compound and the solvent. Therefore, the measured pKa may vary in different solvents.
Regarding basic compounds, the analogous ionization constant Kb is denoted by the equation: Kb = [BH⁺] × [OH⁻] / [HB]. For ease of comparison and calculation, the term pKb is used to represent the strength of a base and is defined as: pKb = -log(Kb). Therefore, stronger basicity is suggested by lower pKb values, remaining consistent with the inverse relationship noted above.
For any conjugate acid-base pair, the sum of the pKa and pKb is constant under specific conditions, often represented as: pKa + pKb = pKw = 14 (at 25°C). Although the pKa is predominantly used to denote acidity, in certain cases, it may be used to denote basicity. However, in this context, the pKa value does not represent the base itself. Instead, it is used to denote the strength of the corresponding conjugate acid. As the pKa value of the conjugate acid increases, it signifies stronger basicity in the form of lower pKb values.
The situation becomes twofold for amphoteric molecules like aniline, which exhibit both acidic and basic properties. When it dissociates to produce H⁺, it signifies its pKa as an acid. However, when it operates as a base, its pKa is representative of its conjugate acid.
Finally, it is crucial to remark that when the concentrations of ionic and non-ionic constituents within a solution are equal (i.e., each consists of 50% of the total), the pH of the solution will be equivalent to the pKa of the molecule in solution.
Nucleotide and Nucleic Acid (DNA/RNA) Isoelectric Point
Isoelectric Point of Nucleotides
Nucleotides, the integral components constituting nucleic acids, comprise deoxyribonucleotide triphosphates (dNTPs) and ribonucleoside triphosphates (NTPs). Various chemical functional groups present within these nucleotides (for example, hydroxyl groups, nitrogenous bases, and phosphate groups) can undergo ionization under varying pH conditions, releasing or absorbing H⁺ ions, thereby leading to alterations in the nucleotides' charged state. The isoelectric point of a nucleotide is identified as a specific pH value where the amount of positive and negative charges within the nucleotide equate, yielding a net charge of zero.
Phosphate groups constitute a crucial component of nucleotides. The primary dissociation pKa of the phosphate moiety falls within the range of 0.7 to 0.9, while the secondary dissociation pKa ranges from 6.0 to 6.4.
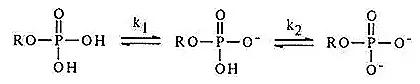
The N atoms and amino groups (NH₂) present on the pyrimidine and purine rings possess the capability to bind and release protons. Under lower pH conditions, these N atoms/amino groups (NH₂) readily absorb a proton (H⁺), transforming into NH₃⁺, exhibiting alkalinity.
As pH gradually increases, the primary dissociation of phosphate groups occurs first (dissociation curve I), followed by the secondary dissociation of phosphate groups (III). Subsequently, the acidic dissociation of hydroxyl groups on pentose sugars initiates (IV), indicating the weakest acidity of the pentose moiety. Concurrently, protonation of nitrogen atoms on the nitrogenous bases gradually diminishes (II).
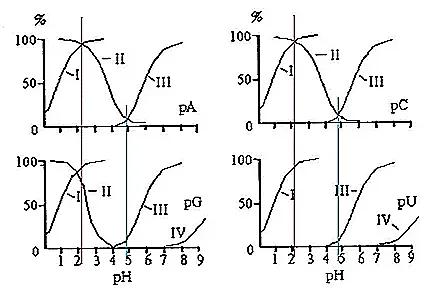
The intersection point of curves I and II represents the isoelectric point, with an approximate pI of 2. At this juncture, the primary dissociation of phosphate groups and the dissociation of bases are precisely balanced. Hence, the negative charges carried by phosphate groups and the positive charges carried by bases are equal. Simultaneously, the secondary dissociation of phosphate groups has not yet occurred.
At pH values below the aforementioned isoelectric point, the degree of primary dissociation of phosphate groups is lower than the degree of protonation of bases, resulting in a net positive charge on nucleotide molecules. Conversely, at pH values above the said isoelectric point but not significantly higher, the degree of primary dissociation of phosphate groups exceeds the degree of protonation of bases, leading to a net negative charge on nucleotide molecules. As pH continues to increase beyond the isoelectric point, the degree of primary dissociation of phosphate groups continues to strengthen, while the dissociation of bases weakens. At a certain moment, concurrent with the enhanced secondary dissociation of phosphate groups (after pH = 5), completion of base deprotonation, and gradual dissociation of the hydroxyl group on the pentose sugar (after pH = 7), these events collectively augment the net negative charge of nucleotide molecules.
Due to the absence of base dissociation, uridine monophosphate (pU) carries a negative charge throughout the entire pH range.
At pH 3.5, the primary dissociation of phosphate groups is complete, resulting in a single negative charge. Different nucleotides exhibit varying degrees of dissociation between their bases, in the order [CMP(+0.84) > AMP(+0.54) > GMP(+0.05) > UMP(+0)], with the strength of negative charge as [CMP < AMP < GMP < UMP]. When non-polar adsorption by the resin is disregarded, the elution sequence is as follows: CMP > AMP > GMP > UMP.
Isoelectric Point of Nucleic Acids
Nucleic acid molecules contain both acidic phosphate groups and weakly basic bases, endowing them with amphoteric properties capable of undergoing amphiprotic dissociation. Given that phosphoric acid exhibits moderate acidity and the bases are weakly basic, the isoelectric point of nucleic acids is comparatively low, typically around 4-4.5 for DNA and 2-2.5 for RNA.
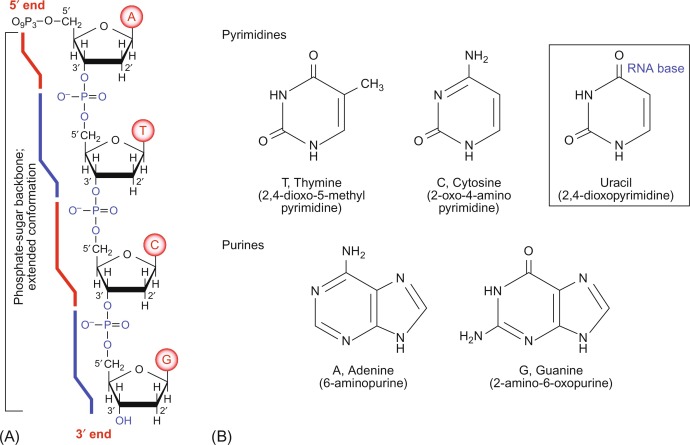
In RNA molecules, the ribose hydroxyl group possesses the capability to form intramolecular hydrogen bonds and can also engage in hydrogen bonding with other molecules such as RNA, proteins, polysaccharides, or the phosphate anions of DNA. Hydrogen bonds represent a distinctive form of intermolecular or intramolecular electrostatic interaction occurring between an electronegative atom X and another electronegative and smaller radius atom Y (such as O or N), forming a structure of X-H...Y, with the hydrogen atom serving as the mediator. When the hydroxyl group of RNA forms intramolecular hydrogen bonds with phosphate anions, it facilitates the ionization of phosphate groups, thereby enhancing the acidity of RNA. In contrast, DNA lacks this effect, hence its acidity is notably lower than that of RNA.
Furthermore, the 2'-OH group in RNA exhibits nucleophilic activity, enabling it to participate in nucleophilic reactions. These reactions can occur not only within RNA molecules but also with other molecules, leading to molecular cleavage. Consequently, RNA molecules are typically smaller in size compared to DNA molecules. Nucleophilic reactions also underlie the catalytic capability of RNA as "ribonucleases," analogous to protein catalytic sites often found in flexible regions (such as loops or unstructured segments) of amino acid side chains bearing hydroxyl groups.
Amino Acid Isoelectric Point
Amino acids, as typical amphoteric molecules, are characterized by the simultaneous presence of acidic carboxyl groups and basic amino groups. How then is theisoelectric point of amino acids calculated? This can be delineated into three distinct scenarios.
Neutral Side Chains
For amino acids containing neutral side chains, they possess two pKa values corresponding to the carboxyl group (pKa1) and the amino group (pKa2). In this scenario, the isoelectric point is the arithmetic mean of these two pKa values, calculated by the formula: pI = (pKa1 + pKa2) / 2. Taking the simplest amino acid, glycine, as an example, with a pKa1 value of 2.34 and a pKa2 value of 9.6, the calculated pI is 5.97.
Acidic Side Chains
In cases where an amino acid features an acidic side chain, the pI tends to occur at a lower pH due to the introduction of additional negative charge. For instance, with aspartic acid, the neutral molecule exhibits a pI between pH 1.88 and 3.65. The calculation for pI involves averaging the pKa values associated with the carboxyl and the third ionizable group, resulting in a pI of 2.77, expressed as: pI = 1/2 (pKa1 + pKa3).
Alkaline Side Chains
When an amino acid harbors an alkaline side chain, the pI tends to occur at a higher pH due to the introduction of additional positive charge. For instance, with histidine, the neutral molecule exists between pH 6.00 and 9.17. The calculation for pI involves averaging the pKa values associated with the second and third ionizable groups, resulting in a pI of 7.59, expressed as: pI = 1/2 (pKa2 + pKa3).
Isoelectric Focusing Electrophoresis Technique
Isoelectric focusing (IEF) electrophoresis is a separation method based on the differential isoelectric point of amphoteric substances. It offers high resolution, capable of distinguishing substances with a difference in pI as small as 0.01, making it an ideal technique for separating amphoteric substances such as proteins.
Principle
The principle of isoelectric focusing involves the establishment of a pH gradient within a gel matrix by incorporating amphoteric electrolytes. During electrophoresis, amphoteric substances concentrate within regions of the gel where the pH equals their respective isoelectric points, thereby facilitating their separation.
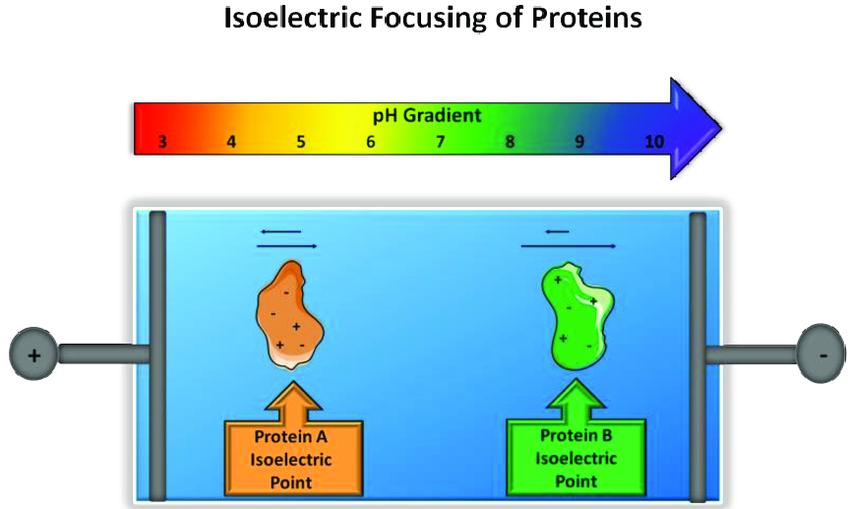
Ampholytes
Ampholytes are complex synthetic mixtures of polyamino and polycarboxyl groups. Various ampholytes possess different pH gradient ranges, encompassing broader spectra such as pH 3-10, as well as narrower ranges like pH 7-8. Selecting appropriate ampholytes according to the characteristics of the sample to be separated is crucial, ensuring that all components of the sample fall within the pH range of the ampholytes. Smaller pH ranges of ampholytes correspond to higher resolution.
Electrophoresis Gels
In isoelectric focusing, horizontal flatbed electrophoresis is commonly employed. Given that isoelectric focusing necessitates the free migration of proteins based on their charge properties within an electric field, lower concentrations of polyacrylamide gels (e.g., 4%) are typically used to prevent molecular sieving. Agarose gels are also frequently utilized, especially for proteins of high molecular weight.
To prepare thin-layer isoelectric focusing gels, ampholytes, riboflavin, and acrylamide are first mixed and poured onto glass plates with spacer bars. Another glass plate is then placed on top to form a flat gel layer.
After polymerization via exposure to light, one glass plate is gently removed, and a small piece of wet filter paper is placed on each side of the gel. Electrode buffer solutions (acidic for the anode, e.g., phosphoric acid solution, and basic for the cathode, e.g., sodium hydroxide solution) are connected to the gel. Upon powering the system, substances with different isoelectric point within the ampholytes undergo electrophoresis in the gel, creating a pH gradient from the anode side to the cathode side, with a linear distribution of pH values increasing from low to high.
Electrophoretic Separation Process
During sample loading, a small piece of filter paper is used to absorb the sample, which is then placed onto the gel. After applying an electric current for 30 minutes, the sample migrates from the filter paper into the gel via electrophoresis, at which point the filter paper can be removed. Initially, the charge carried by the proteins in the sample depends on the pH of the gel where the sample is placed. Proteins with isoelectric points above the gel's pH carry a positive charge and migrate towards the cathode under the influence of the electric field. As the proteins migrate, the pH of the gel surrounding them gradually increases, causing the positive charge carried by the proteins to decrease. When the proteins reach the region of the gel where pH equals their pI, they become neutral and cease migration.
Similarly, proteins with isoelectric points below the pH of the gel where the sample is applied carry a negative charge and migrate towards the anode, eventually halting in the region of the gel where pH equals their pI. It is evident from the isoelectric focusing process that regardless of where the sample is applied on the gel, proteins of various kinds can migrate towards their respective isoelectric points and ultimately halt there, without affecting the final electrophoretic results. Therefore, sometimes samples can be directly added to the gel solution before gel preparation.
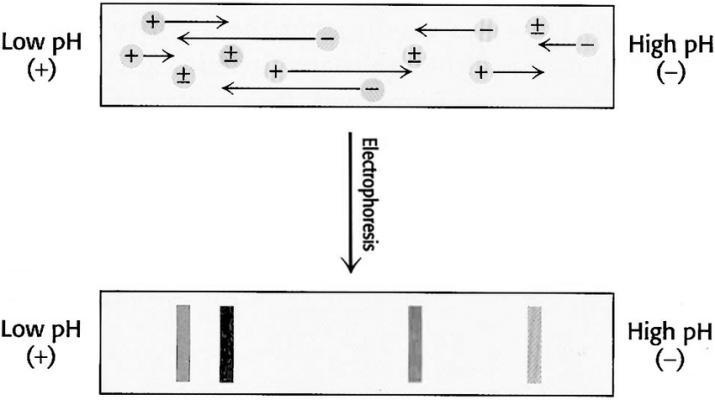
Staining
When staining proteins after the gel electrophoresis process, it's essential to be mindful that ampholytes can also be stained, resulting in the entire gel being stained. Therefore, gels used for isoelectric focusing cannot be directly stained. Prior to staining, they must first undergo immersion in 10% trichloroacetic acid (TCA) to remove the ampholytes.
Applications of Isoelectric Focusing in Protein Research
Isoelectric focusing finds utility in determining the pI of unknown proteins. This is accomplished by subjecting a series of known standard proteins, alongside the protein of interest, to isoelectric focusing simultaneously. The distances migrated by individual bands of the standard proteins to the edge of the gel during electrophoresis are plotted against their respective pI values, thereby generating a standard curve. Subsequently, the distance migrated by the protein of interest is determined, and its pI is calculated using the standard curve.
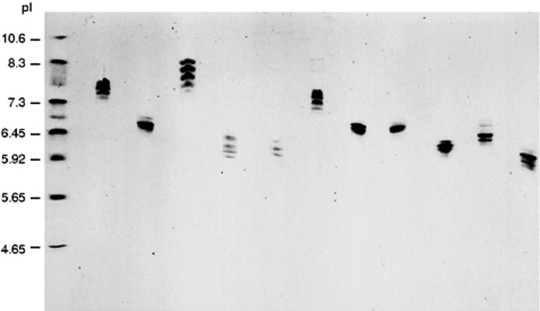
IEF boasts high sensitivity, making it particularly suitable for investigating the micro-heterogeneity of proteins. For instance, a protein may exhibit a single band in SDS-polyacrylamide gel electrophoresis (SDS-PAGE) but present three bands in isoelectric focusing. This phenomenon may stem from the presence of mono-, di-, and tri-phosphorylated forms of the protein. Since several phosphorylation groups do not significantly affect the molecular weight of the protein, it appears as a single band in SDS-PAGE. However, owing to the differing charges they confer, these forms can be separated and detected via isoelectric focusing.
Isoelectric focusing can also yield satisfactory separation results for enzymes with only one or two amino acid differences, such as isoenzymes. As proteins are typically in their native state during isoelectric focusing, enzyme detection can be performed using activity staining methods. While primarily employed for separation analysis, isoelectric focusing can also serve purification purposes.