1. Introduction
The development of circular dichroism (CD) spectroscopy can be traced back to the early 19th century when scientists such as Biot, Fresnel, and Cotton first observed the phenomenon. With advancements in technology, the scope of its applications has expanded significantly. CD spectroscopy holds paramount importance in scientific research as a powerful tool for investigating the structure and conformation of biomacromolecules. It provides crucial insights into secondary structures and conformational changes of molecules. In biopharmaceutical quality control, CD spectroscopy facilitates the determination of secondary structure, stability, and assembly of therapeutic proteins. Additionally, disease diagnostics aids in elucidating the relationship between biomolecules and pathological mechanisms. Due to its unique spectroscopic properties, CD spectroscopy has enabled scientists to explore the microscopic world with greater precision, contributing significantly to life sciences, chemistry, and materials science.
This paper aims to provide a comprehensive overview of the applications of CD spectroscopy, with an in-depth analysis of its role and significance across various domains. The discussion begins with an introduction to CD spectroscopy's fundamental principles, including its physical basis, spectral characteristics, and measurement techniques. Following this, the focus shifts to its applications in protein structure analysis, nucleic acid research, drug development, and emerging fields such as nanomaterials and biosensors. A comparative analysis with other structural characterization techniques, including X-ray crystallography and nuclear magnetic resonance (NMR) spectroscopy, highlights the advantages of CD spectroscopy. Finally, the current state of its applications is summarized alongside an outlook on future developments and potential challenges, providing valuable insights for researchers in relevant fields.
Select Service
Support resource
2. Applications of Circular Dichroism Spectroscopy in Protein Structural Analysis
2.1 Determination of Protein Secondary Structure
CD spectroscopy is a powerful tool for characterizing the secondary structure of proteins. The far-UV region (180-260 nm) of CD spectra predominantly reflects signals from the peptide backbone, providing detailed information on protein conformation.
For example, proteins with a high α-helical content exhibit characteristic negative peaks at 208 nm and 222 nm, whereas β-sheet structures present a distinct positive peak at approximately 215 nm. Researchers can quantitatively estimate the proportion of different secondary structural elements within a protein by analyzing these spectral features. CD spectroscopy offers rapid and reliable secondary structure analysis in solution, avoiding the need for complex sample preparation, such as crystallization, making it highly advantageous for studying native protein conformations under physiological conditions.
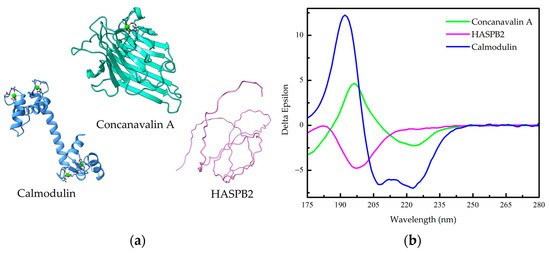
2.2 Protein Folding and Unfolding Studies
CD spectroscopy is widely employed in studying protein folding kinetics and stability. Protein folding involves transitioning from an unstructured polypeptide chain to a well-defined three-dimensional conformation, a process accompanied by structural changes manifesting in CD spectral variations.
Researchers can investigate folding kinetics, intermediate states, and folding pathways by monitoring CD spectra in real time under varying experimental conditions (e.g., temperature, pH, ionic strength). Additionally, CD spectroscopy enables the assessment of protein stability by measuring changes in CD signals upon exposure to denaturing agents, facilitating the determination of protein unfolding temperatures, pH tolerance, and other stability parameters. These insights are critical for understanding protein function, disease mechanisms, and rational drug design.
2.3 Analysis of Protein-Ligand Interactions
CD spectroscopy provides a valuable approach for studying protein-ligand interactions, which are fundamental to many biological processes, including enzyme-substrate binding and receptor-ligand recognition. Ligand binding often induces conformational changes in proteins, which can be detected through CD spectral analysis.
For example, binding a small-molecule ligand to a protein may alter its α-helical content, reflected as changes in CD signals at 208 nm and 222 nm. By monitoring such spectral variations at different ligand concentrations, researchers can determine binding affinities, interaction kinetics, and structural effects of ligand binding. This information is crucial for elucidating molecular recognition mechanisms and optimizing drug design strategies.
Circular dichroism spectroscopy is a valuable tool for investigating the dynamics of protein-ligand interactions. By continuously monitoring CD spectral changes under varying ligand concentrations, it is possible to determine binding kinetics, including the association and dissociation rates and the binding constants. Such information is critical for elucidating the molecular mechanisms underlying protein-ligand interactions, informing drug design, and identifying potential therapeutic targets.
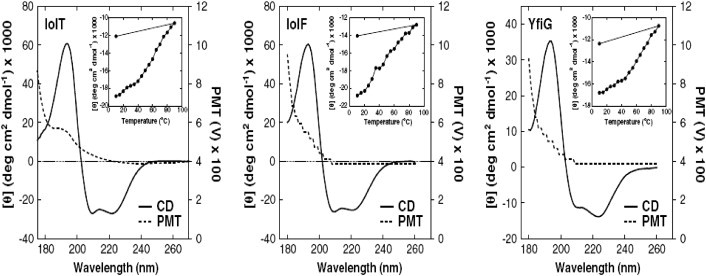
3. Applications of Circular Dichroism Spectroscopy in Nucleic Acid Structural Studies
3.1 Determination of DNA Conformations
CD spectroscopy is a powerful technique for characterizing DNA conformations. In the case of double-helical DNA, B-DNA exhibits characteristic peaks at approximately 248 nm and 275 nm, corresponding to its right-handed helical structure and base stacking interactions, respectively. Changes in solution conditions, such as adding small molecules, can alter peak intensity and position, indicating conformational transitions.
A-DNA, a more compact helical form than B-DNA, is characterized by a positive peak near 260 nm. Corresponding changes in peak intensity and wavelength shifts in the CD spectrum accompany the transition from B-DNA to A-DNA. Z-DNA, a left-handed helical conformation, exhibits a unique CD signature, including a negative peak around 290 nm. Sequences rich in alternating purines and pyrimidines can adopt the Z-DNA conformation under specific conditions, which can be identified using CD spectroscopy.
CD spectroscopy enables precise characterization of DNA conformational changes in response to environmental variations, facilitating in-depth study of structure-function relationships. For example, in drug-DNA interaction studies, CD spectral changes in DNA upon drug binding provide insight into the structural impact of the interaction, informing drug design strategies.
3.2 RNA Structural Studies
CD spectroscopy is also an indispensable tool for RNA structural analysis. RNA secondary structures, including stem loops, hairpins, and pseudoknots, play crucial roles in RNA stability and function. CD spectroscopy effectively captures characteristic spectral changes associated with these structural motifs.
For instance, the formation or disruption of RNA stem-loop structures induces detectable changes in CD signals at specific wavelengths. Analyzing these variations provides insights into RNA secondary structures' stability and conformational dynamics. Furthermore, CD spectroscopy can be employed to investigate functional RNA conformational changes. Riboswitches, for example, undergo structural rearrangements upon ligand binding, and CD spectroscopy can detect these alterations, thereby elucidating RNA functional mechanisms.
CD spectroscopy is also useful in RNA-protein interaction studies. CD spectral shifts can assess RNA secondary structure modifications upon protein binding, offering critical data for understanding RNA-protein complexes. This technique provides valuable perspectives in RNA structural biology, advancing our comprehension of RNA functions and mechanisms in biological systems.
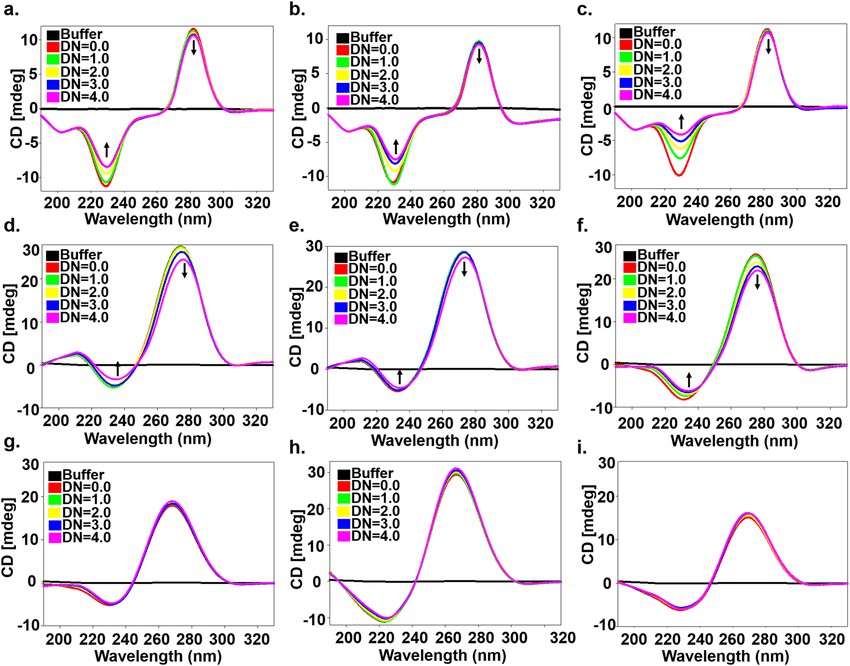
3.3 Analysis of Nucleic Acid-Small Molecule Interactions
CD spectroscopy offers distinct advantages in studying nucleic acid-small molecule interactions, particularly in drug discovery. Ligand binding can induce conformational changes in nucleic acids, which are reflected in the CD spectra.
Achiral small molecules may acquire induced CD (ICD) signals upon interacting with nucleic acids. Analysis of ICD spectra provides crucial information regarding binding sites, modes, and interaction strengths. For instance, corresponding CD spectral shifts can be observed if a small molecule induces a conformational transition from B-DNA to A-DNA or Z-DNA.
CD spectroscopy is a rapid and sensitive tool in drug screening applications. By comparing CD spectra of nucleic acids in the presence of various small molecules, candidates that significantly alter nucleic acid conformation can be identified for further pharmacological evaluation. This approach enhances drug screening efficiency and supports the development of novel therapeutics by elucidating interaction mechanisms and identifying binding targets.
4. Applications of Circular Dichroism Spectroscopy in Chiral Drug Analysis
4.1 Identification of Chiral Drug Isomers
Many pharmaceutical compounds exist as chiral isomers, which can exhibit significantly different pharmacological and toxicological properties. CD spectroscopy is a key method for identifying and characterizing chiral drug isomers.
Chiral molecules exhibit differential absorption of left- and right-circularly polarized light, resulting in distinct CD spectra. For example, Schiff base metal complexes display Cotton effects with opposite signs depending on their R—or S-configuration. The chiral configuration of a drug molecule can be determined by comparing its CD spectrum to reference spectra of known enantiomers.
In practical applications, drug samples are typically dissolved in an appropriate solvent and subjected to CD spectral scanning across relevant wavelength ranges. Analysis of peak positions, intensities, and shapes enables the determination of enantiomeric identity. This approach's rapid, sensitive, and accurate nature ensures its utility in pharmaceutical research, production, and quality control, safeguarding drug efficacy and safety.
4.2 Purity Assessment of Chiral Drugs
CD spectroscopy is crucial in assessing drug purity during production and quality control. Impurities, particularly chiral contaminants, can significantly alter a drug's pharmacological profile, necessitating precise purity evaluation.
Achiral impurities may reduce or eliminate CD signals, whereas chiral impurities may introduce additional spectral features. Drug purity can be assessed by establishing reference CD spectra for pure enantiomers by comparing the test sample spectrum with the standard.
This non-destructive technique requires minimal sample quantities, allowing for rapid and accurate purity determination. CD spectroscopy thus supports stringent quality assurance measures, ensuring compliance with pharmaceutical standards and enhancing drug safety.
4.3 Conformational Analysis of Drug Molecules
Depending on environmental conditions, drug molecules can adopt different conformations, influencing their stability and bioactivity. CD spectroscopy is an effective method for monitoring these conformational dynamics.
Chromophores such as peptide bonds and aromatic rings within drug molecules generate specific CD signals upon exposure to circularly polarized light. Environmental factors, including temperature, pH, and solvent polarity, can induce detectable conformational changes via CD spectral variations.
For example, protein-based drugs undergo structural transitions upon thermal perturbation, leading to CD signal alterations in far- and near-UV regions. CD spectroscopy provides insights into protein folding and stability by tracking these changes.
In studies of drug-target interactions, conformational changes induced by ligand binding can be monitored in real-time, offering valuable structural insights for drug optimization. CD spectroscopy thus provides a sensitive, accurate approach for investigating the conformational properties of drug molecules, contributing to drug development and formulation stability studies.
5. Applications of Circular Dichroism Spectroscopy in Materials Science
5.1 Optical Characterization of Chiral Materials
CD spectroscopy is a unique technique for characterizing the optical activity of chiral materials. These materials exhibit differential absorption of circularly polarized light due to their inherent chiral structures.
Preparing chiral materials as solutions and performing CD spectral scans over specific wavelength ranges can provide detailed insights into their conformations and supramolecular structures. Characteristic Cotton effects provide information on molecular configuration, supramolecular twisting, and periodic helical structures.
This technique aids in the rational design, synthesis, and functional characterization of chiral materials, advancing research in materials science.
5.2 Analysis of Nanoscale Chirality
CD spectroscopy is widely employed in studying the structure and properties of nanomaterials. Traditional characterization methods often have limitations in nanoscale chirality, whereas CD spectroscopy provides new insights into chiral surface arrangements and electronic transitions.
Surface-enhanced CD spectroscopy enables the investigation of chiral nanostructures, offering valuable data on their optical properties. The ability to track CD spectral changes under varying conditions allows for exploring chiral nanomaterials' structure-property relationships, facilitating their optimization for diverse applications in nanotechnology and biomedicine.
5.3 Investigation of Optical Activity in Materials
Circular dichroism (CD) spectroscopy is a powerful tool for studying materials' optical activity and chiral properties. Optical activity is a fundamental characteristic of chiral materials, and CD spectroscopy provides a highly sensitive means to detect and analyze this feature.
A typical study first prepares the material of interest into an appropriate spectroscopic sample, followed by CD spectral scanning across a specified wavelength range. Chiral materials exhibit differential absorption of left- and right-circularly polarized light, generating characteristic CD signals. By analyzing these signals, researchers can determine the material's chirality and assess the influence of chiral structures on its optical properties.
For instance, non-chiral metallic nanoparticles can develop strong CD signals upon interaction with naturally occurring chiral biomolecules. When employed as chiral templates, amyloid fibrils can induce specific chiral morphologies in gold nanorods, simultaneously leading to pronounced CD signals. In complex chiral material systems, CD spectroscopy enables the deconvolution of individual component contributions to the overall CD signal, thereby elucidating the material's optical activity and chiral nature. Such insights lay a theoretical foundation for developing chiral materials with unique optical properties, expanding their applications in optics, electronics, and related fields.
6. Comparison of Circular Dichroism Spectroscopy with Other Structural Analysis Techniques
6.1 Advantages in Sample Requirements
CD spectroscopy offers significant advantages in terms of sample preparation. The process is relatively straightforward, typically requiring only dissolution of the sample in an appropriate solvent at a suitable concentration. For example, protein samples can be readily dissolved in buffer solutions and adjusted to an appropriate CD-analysis concentration. CD spectroscopy imposes fewer purity constraints than X-ray crystallography, which necessitates highly pure and well-crystallized samples.
Furthermore, CD spectroscopy requires only minimal sample quantities. For example, CD measurements in nucleic acid research typically require only microgram-level samples, whereas other structural techniques may necessitate significantly more significant amounts. This is particularly advantageous for rare or difficult-to-obtain samples, allowing researchers to maximize the use of limited resources. Additionally, CD spectroscopy is non-destructive, permitting the recovery and reuse of samples for further experimental investigations, thereby enhancing overall sample efficiency.
6.2 Resolution Comparison with X-ray Crystallography
CD spectroscopy and X-ray crystallography each possess unique advantages in terms of resolution. X-ray crystallography provides ultra-high-resolution structural information at the atomic level, offering precise insights into molecular architecture, including atomic distances and bond angles. This unparalleled resolution renders X-ray crystallography indispensable for elucidating the delicate structures of biomolecules such as proteins and nucleic acids.
In contrast, CD spectroscopy excels in analyzing secondary and tertiary structures, offering sensitivity to conformational changes such as α-helices and β-sheets in proteins. Similarly, for nucleic acids, CD spectroscopy effectively differentiates structural conformations under varying conditions. While CD spectroscopy does not achieve atomic-level resolution, it provides valuable conformational insights in solution, closely resembling biomolecular behavior in physiological environments. Moreover, CD spectroscopy is rapid and straightforward, enabling the efficient acquisition of average structural information across a large number of molecules. This capability is particularly beneficial for investigating biomolecular conformational dynamics and functional mechanisms.
6.3 Advantages in Rapid Structural Analysis
CD spectroscopy excels in rapid structural characterization. A complete CD spectral scan can typically be performed within minutes. For example, in protein structural studies, CD spectroscopy enables swift analysis of secondary structural elements and conformational changes by simply introducing the sample solution into a cuvette and setting the instrument parameters.
Compared to other structural techniques, such as nuclear magnetic resonance (NMR) spectroscopy, which requires extensive data acquisition and processing, or X-ray crystallography, which demands complex crystallization procedures, CD spectroscopy offers superior efficiency. This rapid analysis capability is particularly valuable in applications such as high-throughput drug screening, where quick assessment of molecular structural features is essential. Additionally, CD spectroscopy facilitates real-time monitoring of biomolecular conformational changes under varying conditions, capturing transient structural alterations. This dynamic monitoring capability provides crucial insights into biomolecular functions and interactions.
7. Recent Advances in Circular Dichroism Spectroscopy
7.1 Applications in Single-Molecule Research
Recent advancements in CD spectroscopy have enabled its application at the single-molecule level, moving beyond ensemble-averaged measurements. By integrating single-molecule optical imaging techniques with CD detection, researchers can now explore nanoscale structural dynamics with unprecedented detail.
For proteins, single-molecule CD spectroscopy enables real-time observation of conformational transitions under different environmental conditions, such as folding and unfolding processes. Similarly, in nucleic acid research, this approach allows dynamic monitoring of structural changes during biological processes such as replication and transcription, thereby elucidating functional mechanisms. In nanomaterial studies, single-molecule CD spectroscopy provides insights into the chiral properties of individual nanotubes, nanowires, and other nanostructures, enhancing our understanding of the relationship between chirality and optical properties. These developments offer novel methodologies for investigating the intricate interplay between molecular structure and function across diverse scientific disciplines, including biology, chemistry, and materials science.
7.2 Development of High-Sensitivity CD Techniques
Enhancing the sensitivity of CD spectroscopy has become a primary research focus, leading to significant technological breakthroughs and expanding its applications.
Optimizations in optical design, light source intensity, and detector performance have substantially improved CD detection sensitivity. For instance, the implementation of high-power laser sources in conjunction with highly sensitive photomultiplier tube detectors has facilitated the measurement of extremely weak optical signals, enabling the precise characterization of low-concentration samples and subtle structural variations.
These high-sensitivity CD techniques have broad implications for biomedical research. Disease biomarker detection, for example, enables rapid and accurate quantification of trace-level biomarkers, supporting early disease diagnosis and therapeutic monitoring. In drug development, high-sensitivity CD spectroscopy provides detailed insights into drug-target interactions and in vivo conformational changes, contributing to rational drug design and optimization. Additionally, in materials science, the ability to detect minute chiral variations at the microscale aids the development of advanced chiral materials with tailored optical functionalities. As these technologies continue to evolve, their impact on scientific research and technological innovation is expected to expand significantly.
7.3 Applications in In-Situ Analysis
CD spectroscopy has demonstrated exceptional utility in in situ monitoring of molecular structures and dynamic processes, offering unique advantages in real-time analysis.
In biochemical research, in situ, CD spectroscopy enables real-time tracking of enzyme-substrate interactions and conformational changes during enzymatic reactions. For instance, in protease-catalyzed protein hydrolysis, CD spectroscopy can capture secondary structural alterations, providing mechanistic insights into enzymatic activity. In cell biology, this technique facilitates in situ monitoring of biomolecular structural changes within living cells, advancing our understanding of cellular signaling and gene expression dynamics.
In materials science, CD spectroscopy is employed to monitor structural changes during material synthesis, processing, and application. For example, during the synthesis of chiral materials, in situ CD spectroscopy enables real-time observation of chiral structure formation, guiding the optimization of synthesis parameters. Additionally, in performance evaluation, CD spectroscopy aids in assessing material stability under varying environmental conditions, shedding light on structure-property relationships.
8. Conclusions and Future Perspectives
8.1 Summary of Current Applications
CD spectroscopy has established itself as a versatile analytical technique with widespread applications across multiple disciplines. In biological research, it has provided precise structural characterization of proteins and nucleic acids, elucidating biomolecular functional mechanisms. In pharmaceutical sciences, CD spectroscopy supports chiral drug analysis, purity assessment, and conformational studies, facilitating drug discovery and quality control. In materials science, it plays a crucial role in characterizing chiral materials, thereby driving advancements in nanotechnology and optical material development.
8.2 Future Prospects and Challenges
The future of CD spectroscopy lies in advancements toward higher sensitivity, improved resolution, and real-time dynamic monitoring, with potential applications in single-molecule and live-cell studies. However, challenges remain, including enhancing detection limits for weak signals, optimizing data processing algorithms for complex spectra, and developing new instrumentation compatible with diverse sample types and environments. Overcoming these challenges will unlock the full potential of CD spectroscopy, fostering novel discoveries and technological innovations across various scientific domains.
References
- Paramita, V.D.; Panyoyai, N.; Kasapis, S. Molecular Functionality of Plant Proteins from Low- to High-Solid Systems with Ligand and Co-Solute. Int. J. Mol. Sci. 2020, 21, 2550. https://doi.org/10.3390/ijms21072550
- Crain AV, Broderick JB. Flavodoxin cofactor binding induces structural changes that are required for protein-protein interactions with NADP(+) oxidoreductase and pyruvate formate-lyase activating enzyme. Biochim Biophys Acta. 2013 Dec;1834(12):2512-9. DOI: 10.1016/j.bbapap.2013.08.014. Epub 2013 Sep 7. PMID: 24016774; PMCID: PMC4012331.
- Shahabadi N, Kashanian S, Darabi F. DNA binding and DNA cleavage studies of a water soluble cobalt(II) complex containing dinitrogen Schiff base ligand: the effect of metal on the mode of binding. Eur J Med Chem. 2010 Sep;45(9):4239-45. DOI: 10.1016/j.ejmech.2010.06.020. Epub 2010 Jun 17. PMID: 20598781.
- Hutterer, K.M., Ip, A., Kuhns, S. et al. Analytical Similarity Assessment of ABP 959 in Comparison with Eculizumab Reference Product. BioDrugs 35, 563–577 (2021). https://doi.org/10.1007/s40259-021-00492-9
- Improved mycobacterial protein production using a Mycobacterium smegmatis groEL1ΔCexpression strain | BMC Biotechnology | Full Text. https://bmcbiotechnol.biomedcentral.com/articles/10.1186/1472-6750-11-27
- Linhares, L.A.; Ramos, C.H.I. Unlocking Insights into Folding, Structure, and Function of Proteins through Circular Dichroism Spectroscopy—A Short Review. Appl. Biosci. 2023, 2, 639-655. https://doi.org/10.3390/applbiosci2040040