1. Introduction
1.1 Why Circular Dichroism (CD) Matters in Protein Research
Proteins play a central role in biological processes, with their structure directly linked to function. Understanding protein structure is crucial for studying disease mechanisms, drug development, and biopharmaceutical engineering. Circular Dichroism (CD) spectroscopy is a powerful tool that analyzes protein structures by measuring their differential absorption of left- and right-circularly polarized light.
CD spectroscopy provides key insights into both secondary and tertiary structures, helping scientists track protein folding, stability, and conformational changes under various conditions. This makes CD an essential technique across biological research and pharmaceutical development, offering a direct link between protein structure and function.
1.2 FUV-CD vs. NUV-CD: Key Differences
CD spectroscopy is divided into two primary regions based on wavelength:
- Far-UV CD (FUV-CD, 180–250 nm): Analyzes the peptide bonds in proteins, revealing secondary structures such as alpha-helices, beta-sheets, and random coils.
- Near-UV CD (NUV-CD, 250–320 nm): Focuses on the environment surrounding aromatic amino acids (tryptophan, tyrosine, phenylalanine), providing information on the tertiary structure of proteins.
While FUV-CD excels at detecting secondary structure composition, NUV-CD captures subtle conformational changes in protein folding, ligand binding, and stability. Together, these methods offer a comprehensive approach to studying protein architecture.
2. Far-UV Circular Dichroism (FUV-CD): Principles and Applications
2.1 How FUV-CD Works
Far-UV Circular Dichroism (FUV-CD) is based on the interaction between peptide bonds and circularly polarized light in the far-UV range (180–250 nm). Since proteins have chiral structures, they absorb left- and right-circularly polarized light differently, generating characteristic CD spectra.
- Alpha-helices produce two distinct negative peaks at 208 nm and 222 nm.
- Beta-sheets exhibit a positive peak near 195 nm and a negative peak around 215 nm.
- Random coils show a broad negative peak near 200 nm.
By analyzing these spectral patterns, researchers can estimate the proportion of different secondary structures within a protein, track structural changes, and study protein folding dynamics.
2.2 Measuring FUV-CD: Experimental Setup
FUV-CD measurements require a CD spectropolarimeter, which consists of:
- A stable UV light source
- A monochromator to select specific wavelengths
- A polarizer and modulator to generate circularly polarized light
- A sample chamber with a quartz cuvette (typically 0.1–1 cm path length)
- A detector to record absorption differences
Sample Preparation Considerations:
- Protein concentration: 0.1–1 mg/mL
- Solvent selection: Heavy water (D₂O) is preferred, as standard water absorbs strongly in the far-UV range
- pH and temperature control: Ensures stability during measurements
During the experiment, CD spectra are recorded across 180–250 nm, capturing protein secondary structure composition.
2.3 Advantages and Limitations of FUV-CD
Advantages:
- Rapid and sensitive method for detecting protein structural changes
- Requires minimal sample preparation, making it ideal for limited protein quantities
- Allows real-time monitoring of protein folding, stability, and ligand interactions
Limitations:
- Strong solvent absorption at far-UV wavelengths necessitates careful buffer selection (e.g., D₂O instead of H₂O)
- Cannot provide atomic-resolution structural details, requiring complementary techniques (e.g., X-ray crystallography or NMR)
3. Near-UV Circular Dichroism (NUV-CD): Probing Tertiary Structure
3.1 How NUV-CD Works
Near-UV Circular Dichroism operates in the 250–320 nm range, where the aromatic amino acids (tryptophan, tyrosine, phenylalanine) and disulfide bonds absorb circularly polarized light. The resulting spectral patterns reflect the microenvironment of these residues, providing insights into protein tertiary structure.
- Tryptophan residues are highly sensitive to conformational shifts, making them key indicators of protein folding.
- Tyrosine and phenylalanine contribute additional spectral features, depending on their hydrogen bonding and solvent exposure.
- Disulfide bonds influence CD signals by stabilizing protein folding patterns.
When a protein undergoes conformational changes (e.g., ligand binding, pH shifts, or mutations), its NUV-CD spectrum shifts, revealing structural modifications.
3.2 Experimental Setup for NUV-CD
NUV-CD follows a similar methodology as FUV-CD but operates at longer wavelengths.
Key differences in setup:
- Protein concentrations are typically higher (0.5–2 mg/mL) due to weaker NUV-CD signals.
- Solvent absorption is lower, allowing standard aqueous buffers instead of D₂O.
- Sample handling must minimize external chromophores (e.g., cofactors, dyes) to avoid spectral interference.
3.3 Advantages and Limitations of NUV-CD
Advantages:
- Directly detects tertiary structure stability and conformational shifts
- Compatible with standard aqueous buffers, reducing experimental complexity
- Provides real-time monitoring of protein folding and drug-protein interactions
Limitations:
- Weaker signals compared to FUV-CD, requiring higher protein concentrations
- Limited resolution—unable to pinpoint specific atomic-level interactions without supplementary techniques (e.g., fluorescence spectroscopy or molecular docking)
Comparing Far-UV and Near-UV Circular Dichroism in Protein Analysis
Fundamental Principles and Measurement Methods
FUV-CD and NUV-CD share common optical principles but serve distinct analytical purposes in protein research.
Both techniques rely on the differential absorption of left- and right-circularly polarized light by optically active protein components. However, their spectral focus differs:
- FUV-CD (180–250 nm): Primarily detects the optical activity of peptide bonds, providing insights into secondary structure elements such as α-helices and β-sheets.
- NUV-CD (250–320 nm): Measures the circular dichroism signals from aromatic amino acid side chains (e.g., tryptophan, tyrosine, phenylalanine), making it useful for analyzing tertiary structure changes.
In terms of instrumentation, both techniques utilize a circular dichroism spectropolarimeter with similar core components, including a light source, monochromator, polarizer, modulator, and detector. However, key differences in sample preparation and measurement conditions exist:
- FUV-CD: Requires deuterium oxide (D₂O) as a solvent due to water's strong absorption in the far-UV region. Typical sample concentrations range from 0.1–1 mg/mL, with optical path lengths of 0.1–1 cm and a scanning range of 180–250 nm.
- NUV-CD: Less affected by water absorption, allowing the use of standard aqueous buffers. Samples are typically 0.5–2 mg/mL, with optical path lengths of 1–2 cm and a scanning range of 250–320 nm.
These distinctions highlight the unique technical considerations for each method, making them complementary tools in protein structural studies.
Differences in Protein Structure Analysis
The primary difference between FUV-CD and NUV-CD lies in the structural level each technique investigates:
FUV-CD: Specializes in secondary structure analysis. It quantifies the proportions of α-helices, β-sheets, and random coils by detecting how these elements absorb circularly polarized light. This makes it invaluable for studying protein folding, conformational changes, and ligand-induced structural shifts.
- Example: During protein folding studies, FUV-CD can track the transition from an unfolded to a structured state in real-time.
NUV-CD: Focuses on tertiary structure dynamics by detecting changes in the local environments of aromatic amino acid residues. These measurements provide insights into protein stability, ligand interactions, and conformational rearrangements.
- Example: In drug discovery, NUV-CD helps assess how ligand binding alters a protein's tertiary structure, offering key data for structure-based drug design.
However, each method has limitations:
- FUV-CD struggles to differentiate between domains in multi-subunit proteins.
- NUV-CD lacks sensitivity for proteins with few aromatic residues.
These factors necessitate careful selection of the appropriate technique based on the specific research question.
Feature | FUV-CD | NUV-CD |
Wavelength Range | 180-250 nm | 250-320 nm |
Main Target | Peptide bonds (secondary structure) | Aromatic residues (tertiary structure) |
Structural Information | Alpha-helix, beta-sheet content | Protein folding, ligand binding effects |
Solvent | Deuterium oxide (D₂O) | Water (H₂O) |
Sample Concentration | 0.1-1 mg/mL | 0.5-2 mg/mL |
Advantages | High sensitivity, no labeling required | Provides microenvironment data, cost-effective |
Limitations | Water interference, struggles with complex domains | Low sensitivity for proteins lacking aromatic residues |
Complementary Use in Protein Research
Given their distinct analytical strengths, combining FUV-CD and NUV-CD offers a more comprehensive view of protein structure and dynamics:
Protein Folding Studies:
- FUV-CD tracks real-time secondary structure formation.
- NUV-CD monitors changes in aromatic residue environments as the protein assumes its native fold.
Ligand Binding & Drug Discovery:
- FUV-CD assesses ligand-induced secondary structure alterations.
- NUV-CD detects conformational shifts in binding sites, aiding in drug optimization.
Multi-Domain Protein Analysis:
- FUV-CD reveals domain-level folding events.
- NUV-CD provides information on the relative positioning of aromatic side chains.
By leveraging both techniques, researchers can obtain a multi-layered understanding of protein behavior, driving advancements in biopharmaceuticals, structural biology, and precision medicine.
You May Be Interested In
Learn More
Case Study: FUV-CD in Protein Structure Research
FUV-CD Unveiling Protein-Polyphenol Interactions
Far-UV Circular Dichroism plays a crucial role in studying protein secondary structure and its dynamic changes under different conditions. One notable application is in understanding how plant proteins interact with polyphenols, such as tea polyphenols and epigallocatechin gallate (EGCG).
Case 1: Structural Regulation of Plant Protein-Polyphenol Complexes
In studies on soybean glycinin and soybean trypsin inhibitor, FUV-CD revealed how polyphenols influence protein conformation. Upon binding with flavonoids, significant changes in the secondary structure were observed:
- Decrease in β-sheet content: The FUV-CD spectrum showed a weakened signal at 217 nm, indicating a reduction in β-sheet structures.
- Increase in random coil content: A stronger signal at 200 nm suggested an increase in disorder, meaning the protein became more flexible.
These findings indicate that polyphenols interact with proteins through hydrogen bonding and hydrophobic interactions, leading to increased protein surface hydrophilicity. Such structural modifications can significantly impact protein functionality, which is particularly relevant in food science and drug formulation.
By leveraging FUV-CD, researchers gain valuable insights into protein stability, folding mechanisms, and bioactive compound interactions, paving the way for improved functional food and pharmaceutical applications.
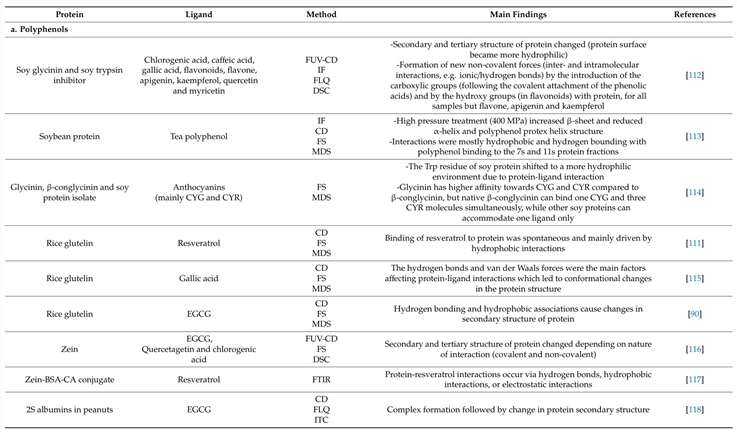
Case 2: Cofactor Binding Mechanism of E. coli Flavodoxin
FUV-CD is a powerful tool for investigating protein-ligand interactions, particularly in understanding how cofactors stabilize protein structure. A key example is its application in studying the cofactor binding mechanism of E. coli Flavodoxin (Fld).
Tracking Structural Changes in Apo-Fld to Holo-Fld Conversion
Flavodoxin exists in two forms:
- Apo-Fld (cofactor-free form)
- Holo-Fld (cofactor-bound form, with flavin mononucleotide (FMN))
FUV-CD spectra revealed significant secondary structure changes during FMN binding:
- Increased α-helix content: The characteristic peaks at 208 nm and 222 nm became more pronounced, indicating α-helix stabilization.
- Stable β-sheet structure: The signal at 217 nm remained unchanged, confirming that β-sheet regions were not significantly altered.
These results suggest that FMN binding primarily enhances α-helical stability, which in turn promotes protein-protein interactions and functional activity.
These two case studies highlight FUV-CD's versatility in studying complex biological systems, from ligand-induced conformational changes to disease-related protein misfolding (e.g., amyloid fibril formation). By providing high-resolution spectral data, FUV-CD offers an indispensable molecular perspective on protein structure-function relationships, aiding in fields such as drug discovery, enzymology, and biophysics.
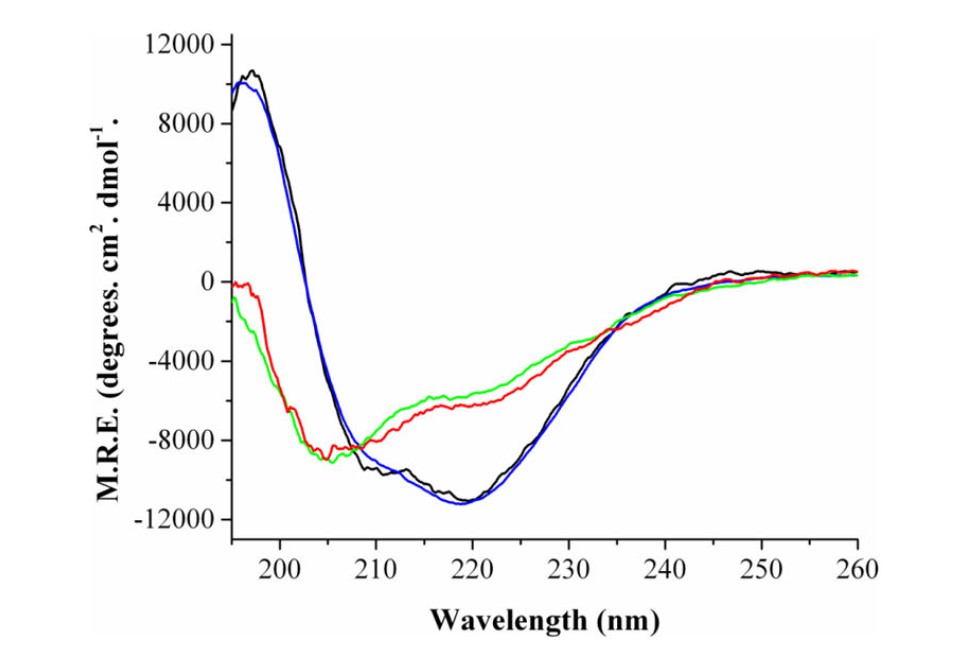
Secondary Structure of E. coli Fld as determined by far-UV CD.
NUV-CD in Protein Structure Research: Key Applications and Insights
Near-ultraviolet circular dichroism is a powerful technique for studying protein tertiary structures. It is especially useful in analyzing how ligand binding induces conformational changes.
One study published in Biochemistry (Liang et al., 2018) used NUV-CD to investigate the interaction between human serum albumin (HSA) and the anti-inflammatory drug ibuprofen. The findings revealed that drug binding alters the microenvironment of aromatic residues, which directly impacts protein stability and function. This insight is crucial for rational drug design, as modifying protein conformation can optimize drug delivery efficiency.
Why Use NUV-CD? Two Key Advantages
1. Microenvironment Sensitivity – NUV-CD detects structural changes in tryptophan, tyrosine, and disulfide bonds, providing real-time insights into ligand-induced conformational shifts.
2. Integration with Other Techniques – It complements fluorescence spectroscopy and molecular docking, bridging the gap between atomic-scale interactions and functional outcomes.
Combining FUV-CD and NUV-CD for Comprehensive Protein Analysis
By integrating far-ultraviolet (FUV-CD) and near-ultraviolet (NUV-CD) techniques, researchers can achieve a multi-level view of protein structure.
A study published in BioDrugs (2021) demonstrated this approach using ABP 959, a biosimilar to the monoclonal antibody Eculizumab, which targets complement protein C5. Researchers used:
- FUV-CD to assess secondary structure (alpha-helices, beta-sheets)
- NUV-CD to evaluate tertiary structure (aromatic residues, disulfide bonds)
- Differential Scanning Calorimetry (DSC) for thermal stability analysis
The results confirmed that ABP 959 and the reference drug Eculizumab had comparable structural integrity, meeting regulatory requirements for biosimilar approval.
Why Combine FUV-CD and NUV-CD?
- Comprehensive Structural Data – Captures changes at both the secondary and tertiary levels.
- Enhanced Drug Development – Ensures biosimilarity in monoclonal antibody (mAb) therapies, reducing clinical trial risks.
Future Trends in CD Spectroscopy
1. High-Throughput CD for Faster Analysis
Advances in automated CD spectroscopy allow for rapid screening of large protein libraries. This is crucial for:
- Accelerating protein drug discovery
- Studying conformational changes in highly dynamic proteins
2. Capturing Protein Dynamics in Real Time
Emerging time-resolved CD (TRCD) can track protein folding on a millisecond to microsecond scale. This helps scientists understand how proteins transition between functional and misfolded states, which is key in disease research (e.g., amyloid disorders).
3. AI-Driven Data Analysis
Artificial intelligence (AI) is revolutionizing CD data interpretation. Machine learning models can:
- Recognize hidden patterns in CD spectra
- Predict secondary and tertiary structure changes
- Improve the accuracy of protein structure modeling
4. Expanding Applications in Drug Discovery
- FUV-CD and NUV-CD aid in screening small-molecule drugs and biologics that interact with proteins.
- Structural insights from CD can guide protein engineering, optimizing stability and efficacy in therapeutic proteins.
Conclusion: The Growing Role of CD Spectroscopy
- FUV-CD and NUV-CD provide complementary insights into protein structure, from backbone folding to ligand-induced microenvironment changes.
- Their integration is critical in biopharma, particularly for biosimilar characterization and rational drug design.
- Future advancements, including high-throughput analysis, AI integration, and real-time structural tracking, will further expand their role in protein science and drug discovery.
By leveraging these techniques, researchers can develop safer, more effective biopharmaceuticals, driving innovation in protein-based therapeutics.
References
- Paramita, V.D.; Panyoyai, N.; Kasapis, S. Molecular Functionality of Plant Proteins from Low- to High-Solid Systems with Ligand and Co-Solute. Int. J. Mol. Sci. 2020, 21, 2550. https://doi.org/10.3390/ijms21072550
- Crain AV, Broderick JB. Flavodoxin cofactor binding induces structural changes that are required for protein-protein interactions with NADP(+) oxidoreductase and pyruvate formate-lyase activating enzyme. Biochim Biophys Acta. 2013 Dec;1834(12):2512-9. DOI: 10.1016/j.bbapap.2013.08.014. Epub 2013 Sep 7. PMID: 24016774; PMCID: PMC4012331.
- Shahabadi N, Kashanian S, Darabi F. DNA binding and DNA cleavage studies of a water soluble cobalt(II) complex containing dinitrogen Schiff base ligand: the effect of metal on the mode of binding. Eur J Med Chem. 2010 Sep;45(9):4239-45. DOI: 10.1016/j.ejmech.2010.06.020. Epub 2010 Jun 17. PMID: 20598781.
- Hutterer, K.M., Ip, A., Kuhns, S. et al. Analytical Similarity Assessment of ABP 959 in Comparison with Eculizumab Reference Product. BioDrugs 35, 563–577 (2021). https://doi.org/10.1007/s40259-021-00492-9
- Improved mycobacterial protein production using a Mycobacterium smegmatis groEL1ΔCexpression strain | BMC Biotechnology | Full Text. https://bmcbiotechnol.biomedcentral.com/articles/10.1186/1472-6750-11-27