Antibody purification is a highly specialized process requiring meticulous optimization at each stage. By leveraging advanced platform technologies and understanding the intricacies of each purification step, including chromatography, loading, washing, and maintenance, the overall efficiency and product quality can be significantly enhanced. Proper documentation and validation strategies further ensure that the processes are scalable and compliant with clinical and regulatory standards.
The primary objectives of antibody purification are as follows:
Process Optimization: Encompasses affinity purification, polishing steps, and viral inactivation/removal processes to enhance purification efficiency and product quality.
Small-Scale Purification: Provides high-purity samples for pharmacological, toxicological, and stability studies, ensuring accuracy and reliability of experimental data.
Process Scale-Up and Pilot Production: Involves scaling up the optimized purification process to meet medium-scale production demands (e.g., 200 L) while ensuring the production of material that meets clinical submission standards.
Documentation: Detailed process documentation is essential to support clinical filings and facilitate smooth technology transfer and subsequent production phases.
Strategies to Improve Antibody Purification Efficiency
Platform Technologies: Platform approaches not only improve purification efficiency but also significantly reduce validation costs. By incorporating the precision and sensitivity of quantitative polymerase chain reaction (Q-PCR), platform technologies provide robust support for evaluating overall impurity and viral clearance efficiency. In these platforms, anion-exchange steps are meticulously designed as polishing stages to effectively remove impurities, such as residual DNA, host cell proteins, and viral particles.
Anion-Exchange Chromatography: Given its widespread use in monoclonal antibody (mAb) purification processes, significant knowledge and expertise have been accumulated in this domain. It is noteworthy that most monoclonal antibodies share a similar isoelectric point (pI) greater than 8, making them less prone to binding under neutral pH and low conductivity conditions. Combining high-flow agarose-based anion exchangers with Q-PCR analysis has demonstrated the efficacy of anion-exchange chromatography for removing non-enveloped viruses.
Improving Chromatography Media Longevity: During validation, another promising strategy is optimizing the lifespan of Protein A and anion-exchange chromatography media. Studies using flow-through anion-exchange chromatography have revealed the value of alternative metrics, such as DNA clearance rate, backpressure, and scalability, in predicting declines in viral clearance capacity. Furthermore, during multi-cycle operations of Protein A chromatography columns, key performance quality attributes, such as antibody yield per step and breakthrough volumes (rather than impurity levels in the eluate), have emerged as critical indicators of process robustness. These attributes tend to exhibit signs of decline before any noticeable reduction in the logarithmic reduction value (LRV) of retrovirus particles.
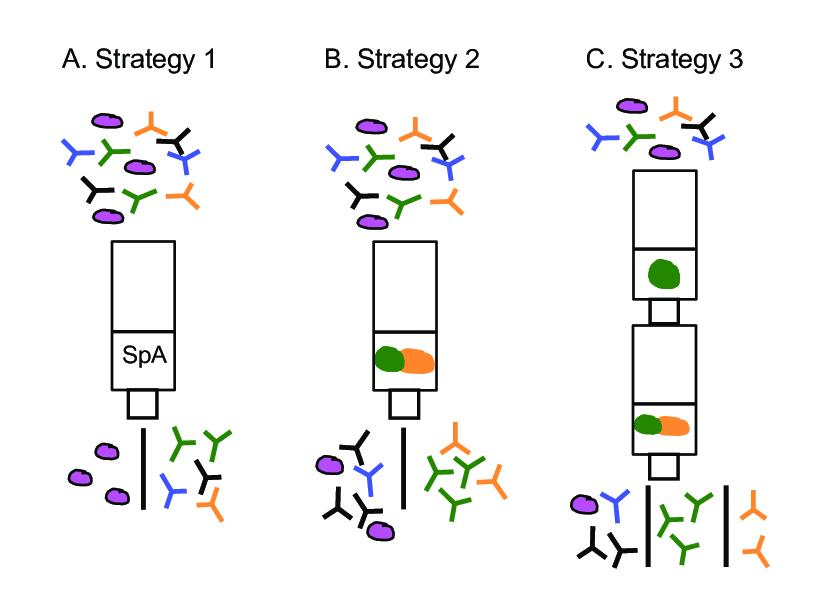
Key Steps in the Chromatographic Purification Process
Equilibration: The objective of this step is to bring the chromatography column to a stable, usable state, ensuring optimal binding conditions and establishing the baseline of the chromatographic curve, which is crucial for subsequent operations.
Loading: During this phase, the target molecules must achieve sufficient, uniform, and limited interaction with the chromatography media to complete the binding process. Key parameters, such as sample volume and loading flow rate, significantly affect separation efficiency and must be optimized through experimentation to identify the ideal loading conditions.
Wash: This step aims to remove any unbound or loosely bound substances from the media surface, pores, and interstitial spaces, thereby enhancing the purity of the separated target molecule.
Elution: In this phase, bound molecules are sequentially eluted from the media, facilitating their separation. The elution process can be divided into multiple steps if necessary to separate molecules with differing binding affinities to the chromatography media.
Maintenance: Maintenance includes the regeneration, cleaning, disinfection, and storage of the chromatography column to ensure long-term operational stability and reuse performance.
Select Service
Learn More
- Application of Mass Spectrometry in Antibody Drug Development: Disulfide Bond Analysis
- Analysis Strategies of Antibody Charge Variants
- Introduction and Characterization of Monoclonal Antibody Drugs
- Application of Mass Spectrometry Technology in Antibody Drug Analysis
- Analytical Methods for Antibody-Drug Conjugates (ADCs)
- Mass Spectrometry-based Antibody De Novo Sequencing: Applications, Advances, and Approaches
Common Methods for Clarification of Fermentation Broth
Clarification of fermentation broth primarily relies on technologies such as depth filtration, tangential flow filtration (TFF), and continuous flow centrifugation. These methodologies are crucial in downstream processing, particularly in large-scale biologics production.
Depth Filtration: This technique is widely used due to its simplicity, ease of implementation, and relatively low initial investment. Depth filters feature a broad pore size distribution and a large internal surface area, allowing for efficient removal of particulate matter through both size exclusion and adsorption mechanisms. In comparison to single-pore filters, depth filtration systems exhibit higher throughput and faster flow rates. Typically, these systems consist of multistage filters with progressively decreasing pore sizes. However, it is important to note that depth filtration alone does not provide sterile filtration, and therefore, sterile filters must be included at the final stage. Additionally, diatomaceous earth, a component of some depth filters, can acquire a positive charge under specific conditions, facilitating the removal of negatively charged host cell nucleic acids and proteins alongside cellular debris.
Challenges of Depth Filtration: Despite its advantages, depth filtration has certain limitations. The disposable filter cartridges are costly, and the processing capacity is limited, especially when handling large volumes of culture fluid. This often necessitates the parallel use of multiple filters, which increases operational costs and footprint. Consequently, depth filtration is more suitable for medium-scale antibody purification processes ranging from 100 to 2000 liters.
TFF: TFF is commonly employed in perfusion bioreactor systems. Its high tangential flow rates help minimize cell deposition on the membrane surface, thereby allowing the processing of large volumes of cell culture fluid without significant membrane fouling. However, increasing flow rates, while reducing cell deposition, may elevate the risk of cell rupture. Hollow fiber filters, known for their large surface area and ability to generate high shear forces within the lumen, are particularly favored for scale-up processes. When optimizing TFF, various factors such as filter chemistry, surface area, pore size, flow rate, and transmembrane pressure must be considered. While TFF allows for large-scale processing, it is associated with dead volume, which may lead to product loss. This issue can be mitigated by adding small volumes of buffer to reduce antibody retention. Nevertheless, the high cost of TFF filters, prolonged filtration times, and limited control over filtration speed are important considerations.
Continuous Flow Centrifugation: Continuous flow centrifugation, particularly using disc-stack centrifuges, has emerged as a key separation method in large-scale antibody production. The conical chamber design enables rapid separation of cells from the harvested culture fluid, where the supernatant is expelled while cells accumulate at the base of the cone. This method offers high removal efficiency, large processing volumes, operational simplicity, and low operating costs. However, the high initial capital investment and complex cleaning procedures are notable drawbacks, as is the absence of appropriate small-scale models for process development. Furthermore, the formation of cell aggregates during centrifugation may lead to nutrient deprivation or the accumulation of byproducts. The shear forces generated during centrifugation may also impact cellular integrity, necessitating careful consideration of cell shear tolerance. Nonetheless, continuous flow centrifugation remains a promising technique due to its high-speed separation and capacity to handle large volumes.
Common Purification Methods in Polishing Steps
Several chromatographic techniques are employed during the polishing stages of antibody purification to remove impurities and ensure product quality:
Anion Exchange Chromatography: Primarily aimed at removing host cell proteins (HCPs) and host nucleic acids.
Cation Exchange Chromatography: Focused on the removal of aggregates, residual HCPs, and Protein A.
Hydrophobic Interaction Chromatography (HIC): Used for the elimination of aggregates, HCPs, and Protein A.
Hydroxyapatite Chromatography and Size-Exclusion Chromatography: These methods are utilized to remove HCPs, Protein A, aggregates, and antibody fragments.
It is important to note that anion exchange chromatography and hydrophobic interaction chromatography typically employ a flow-through mode, where impurities pass through the column without binding, whereas cation exchange chromatography and hydroxyapatite chromatography operate in a bind-and-elute mode, allowing for selective binding and controlled elution of target molecules.